
- •Contents
- •Preface to the first edition
- •Flagella
- •Cell walls and mucilages
- •Plastids
- •Mitochondria and peroxisomes
- •Division of chloroplasts and mitochondria
- •Storage products
- •Contractile vacuoles
- •Nutrition
- •Gene sequencing and algal systematics
- •Classification
- •Algae and the fossil record
- •REFERENCES
- •CYANOPHYCEAE
- •Morphology
- •Cell wall and gliding
- •Pili and twitching
- •Sheaths
- •Protoplasmic structure
- •Gas vacuoles
- •Pigments and photosynthesis
- •Akinetes
- •Heterocysts
- •Nitrogen fixation
- •Asexual reproduction
- •Growth and metabolism
- •Lack of feedback control of enzyme biosynthesis
- •Symbiosis
- •Extracellular associations
- •Ecology of cyanobacteria
- •Freshwater environment
- •Terrestrial environment
- •Adaption to silting and salinity
- •Cyanotoxins
- •Cyanobacteria and the quality of drinking water
- •Utilization of cyanobacteria as food
- •Cyanophages
- •Secretion of antibiotics and siderophores
- •Calcium carbonate deposition and fossil record
- •Chroococcales
- •Classification
- •Oscillatoriales
- •Nostocales
- •REFERENCES
- •REFERENCES
- •REFERENCES
- •RHODOPHYCEAE
- •Cell structure
- •Cell walls
- •Chloroplasts and storage products
- •Pit connections
- •Calcification
- •Secretory cells
- •Iridescence
- •Epiphytes and parasites
- •Defense mechanisms of the red algae
- •Commercial utilization of red algal mucilages
- •Reproductive structures
- •Carpogonium
- •Spermatium
- •Fertilization
- •Meiosporangia and meiospores
- •Asexual spores
- •Spore motility
- •Classification
- •Cyanidiales
- •Porphyridiales
- •Bangiales
- •Acrochaetiales
- •Batrachospermales
- •Nemaliales
- •Corallinales
- •Gelidiales
- •Gracilariales
- •Ceramiales
- •REFERENCES
- •Cell structure
- •Phototaxis and eyespots
- •Asexual reproduction
- •Sexual reproduction
- •Classification
- •Position of flagella in cells
- •Flagellar roots
- •Multilayered structure
- •Occurrence of scales or a wall on the motile cells
- •Cell division
- •Superoxide dismutase
- •Prasinophyceae
- •Charophyceae
- •Classification
- •Klebsormidiales
- •Zygnematales
- •Coleochaetales
- •Charales
- •Ulvophyceae
- •Classification
- •Ulotrichales
- •Ulvales
- •Cladophorales
- •Dasycladales
- •Caulerpales
- •Siphonocladales
- •Chlorophyceae
- •Classification
- •Volvocales
- •Tetrasporales
- •Prasiolales
- •Chlorellales
- •Trebouxiales
- •Sphaeropleales
- •Chlorosarcinales
- •Chaetophorales
- •Oedogoniales
- •REFERENCES
- •REFERENCES
- •EUGLENOPHYCEAE
- •Nucleus and nuclear division
- •Eyespot, paraflagellar swelling, and phototaxis
- •Muciferous bodies and extracellular structures
- •Chloroplasts and storage products
- •Nutrition
- •Classification
- •Heteronematales
- •Eutreptiales
- •Euglenales
- •REFERENCES
- •DINOPHYCEAE
- •Cell structure
- •Theca
- •Scales
- •Flagella
- •Pusule
- •Chloroplasts and pigments
- •Phototaxis and eyespots
- •Nucleus
- •Projectiles
- •Accumulation body
- •Resting spores or cysts or hypnospores and fossil Dinophyceae
- •Toxins
- •Dinoflagellates and oil and coal deposits
- •Bioluminescence
- •Rhythms
- •Heterotrophic dinoflagellates
- •Direct engulfment of prey
- •Peduncle feeding
- •Symbiotic dinoflagellates
- •Classification
- •Prorocentrales
- •Dinophysiales
- •Peridiniales
- •Gymnodiniales
- •REFERENCES
- •REFERENCES
- •Chlorarachniophyta
- •REFERENCES
- •CRYPTOPHYCEAE
- •Cell structure
- •Ecology
- •Symbiotic associations
- •Classification
- •Goniomonadales
- •Cryptomonadales
- •Chroomonadales
- •REFERENCES
- •CHRYSOPHYCEAE
- •Cell structure
- •Flagella and eyespot
- •Internal organelles
- •Extracellular deposits
- •Statospores
- •Nutrition
- •Ecology
- •Classification
- •Chromulinales
- •Parmales
- •Chrysomeridales
- •REFERENCES
- •SYNUROPHYCEAE
- •Classification
- •REFERENCES
- •EUSTIGMATOPHYCEAE
- •REFERENCES
- •PINGUIOPHYCEAE
- •REFERENCES
- •DICTYOCHOPHYCEAE
- •Classification
- •Rhizochromulinales
- •Pedinellales
- •Dictyocales
- •REFERENCES
- •PELAGOPHYCEAE
- •REFERENCES
- •BOLIDOPHYCEAE
- •REFERENCE
- •BACILLARIOPHYCEAE
- •Cell structure
- •Cell wall
- •Cell division and the formation of the new wall
- •Extracellular mucilage, biolfouling, and gliding
- •Motility
- •Plastids and storage products
- •Resting spores and resting cells
- •Auxospores
- •Rhythmic phenomena
- •Physiology
- •Chemical defense against predation
- •Ecology
- •Marine environment
- •Freshwater environment
- •Fossil diatoms
- •Classification
- •Biddulphiales
- •Bacillariales
- •REFERENCES
- •RAPHIDOPHYCEAE
- •REFERENCES
- •XANTHOPHYCEAE
- •Cell structure
- •Cell wall
- •Chloroplasts and food reserves
- •Asexual reproduction
- •Sexual reproduction
- •Mischococcales
- •Tribonematales
- •Botrydiales
- •Vaucheriales
- •REFERENCES
- •PHAEOTHAMNIOPHYCEAE
- •REFERENCES
- •PHAEOPHYCEAE
- •Cell structure
- •Cell walls
- •Flagella and eyespot
- •Chloroplasts and photosynthesis
- •Phlorotannins and physodes
- •Life history
- •Classification
- •Dictyotales
- •Sphacelariales
- •Cutleriales
- •Desmarestiales
- •Ectocarpales
- •Laminariales
- •Fucales
- •REFERENCES
- •PRYMNESIOPHYCEAE
- •Cell structure
- •Flagella
- •Haptonema
- •Chloroplasts
- •Other cytoplasmic structures
- •Scales and coccoliths
- •Toxins
- •Classification
- •Prymnesiales
- •Pavlovales
- •REFERENCES
- •Toxic algae
- •Toxic algae and the end-Permian extinction
- •Cooling of the Earth, cloud condensation nuclei, and DMSP
- •Chemical defense mechanisms of algae
- •The Antarctic and Southern Ocean
- •The grand experiment
- •Antarctic lakes as a model for life on the planet Mars or Jupiter’s moon Europa
- •Ultraviolet radiation, the ozone hole, and sunscreens produced by algae
- •Hydrogen fuel cells and hydrogen gas production by algae
- •REFERENCES
- •Glossary
- •Index

CYANOBACTERIA 41
Fig. 2.16 Light micrograph of Anabaena crassa showing
vegetative cells, akinetes, and heterocysts. (From Li et al.,
1997.)
glucose molecules, that is similar to amylopectin (Hough et al., 1952; Frederick, 1951).
Gas vacuoles
A gas vacuole is composed of gas vesicles, or hollow cylindrical tubes with conical ends, in the cytoplasm of cyanobacteria (Figs. 2.11, 2.17) (Walsby, 1994; Oliver, 1994). Gas vesicles do not have true protein-lipid membranes, being composed exclusively of protein ribs or spirals arranged similarly to the hoops on a barrel. It is possible to collapse the gas vesicles by applying pressure to the cells, the collapsed vesicles having the two halves stuck together. The membrane of
the gas vesicle is quite rigid, with the gas inside it at a pressure of 1 atm. The membrane is permeable to gases, allowing the contained gas to equilibrate with gases in the surrounding solution. The membrane must, however, be able to exclude water. It has been postulated that the inner surface must be hydrophobic, thereby preventing condensation on it of water droplets, and restraining, by surface tension, water creeping through the pores. At the same time these molecules must present a hydrophilic surface at the outer (water-facing) surface in order to minimize the interfacial tension, which would otherwise result in the collapse of the gas vacuole.
Cyanobacteria possessing gas vacuoles can be divided into two physiological-ecological groups. In the first group are those algae having vacuoles only at certain stages of their life cycle, or only in certain types of cells. In Gloeotrichia ghosei and in certain
(a) |
(b) |
Fig. 2.17 (a) Transmission electron micrograph of two cells of
Oscillatoria redekei showing a cross wall separating areas of gas vacuoles (gv); (pg) lipid droplet. (From Whitton and Peat, 1969.) (b) Freeze-etch preparation of gas vacuoles. (From Jones and Jost, 1970.)
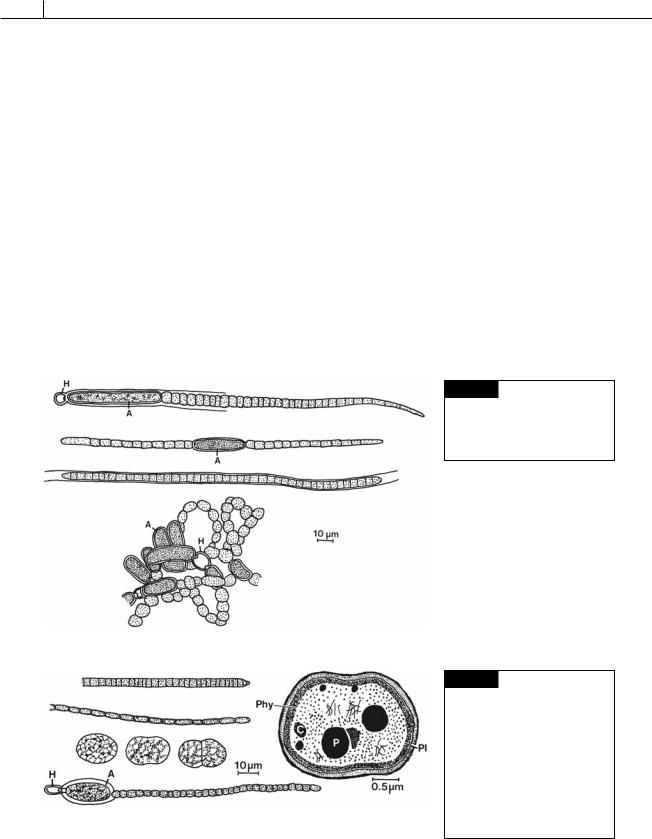
42 THE PROKARYOTIC ALGAE
species of Tolypothrix and Calothrix, gas vesicles appear only in hormogonia. The hormogonia float when they are released, and it is possible that the buoyancy provided is of significance in dispersal of these stages. The second group consists of planktonic cyanobacteria, including species of Anabaena (Figs. 2.16, 2.18(d), 2.57(b)), Gloeotrichia (Fig. 2.18(a)),
Microcystis (Figs. 2.48, 2.56(b)), Aphanizomenon (Fig. 2.18(b)), Oscillatoria (Figs. 2.19(a), (b), 2.34(a), (b)),
Trichodesmium (Figs. 2.31, 2.56(g)), and Phormidium
(Figs. 2.18(c), 2.56(c)). These algae derive positive buoyancy from their gas vesicles, and as a consequence form blooms floating near the water surface. The loss of buoyancy, and subsequent sinking of these algae in the water column, can be due to different factors. In Anabaena flos-aquae (Fig. 2.18(d)), the loss of buoyancy is caused by the loss of gas vesicles owing to increased turgor pressure, whereas in
(a)
(b)
(c)
(d)
Oscillatoria agardhii (Fig. 2.19(a)), buoyancy is lost through cessation of gas vesicle production and an increase in cell mass. In Microcystis aeruginosa (Fig. 2.56(b)), loss of buoyancy can be due to entrapment of whole colonies in a colloidal precipitate composed of organic material and iron salts. The colloidal precipitate is formed in certain lakes when dissolved iron in the anoxic water of the hypolimnion in stratified lakes becomes oxidized on mixing with aerated water of the epilimnion (Oliver et al., 1985).
There is a direct relationship between buoyancy and light quantity in nitrogen-fixing cyanobacteria such as Anabaena flos-aquae (Fig. 2.18(d)) (Spencer and King, 1985). The relationship is complex and also involves the concentration of ammonium ions (NH4 ) in the water. Buoyancy in Anabaena flos-aquae increases under low irradiance (less than 10 E m 2
Fig. 2.18 (a) Gloeotrichia echinulata. (b) Aphanizomenon flosaquae. (c) Phormidium inundatum. (d)
Anabaena flos-aquae. (A) Akinete; (H) heterocyst. (After Prescott,1962.)
(a)
(b)
(c)
(d)
Fig. 2.19 (a) Oscillatoria agardhii. (b) O. limnetica. (c) Synechococcus aeruginosus. (d) Cylindrospermum majus. (A) Akinete; (H) heterocyst. (e) Drawing of the fine structure of Gloeobacter violaceus. (C)
Cyanophycin granule; (P)
(e)
polyphosphate body; (Phy) probable layer of phycobiliproteins; (Pl) plasmalemma. ((c),(d) after Prescott, 1962; (e) after Rippka et al., 1974.)

CYANOBACTERIA 43
s 1), absence of NH4 , and low CO2 concentrations. Such conditions occur in many stagnant eutrophic lakes during the summer. In these lakes, rapid growth of algae has depleted the NH4 and CO2. The water transmits little light because of the large standing crop of algae. Under these conditions, A. flos-aquae and other nitrogen-fixing cyanobacteria having gas vacuoles increase their buoyancy and rise close to the surface of the water. Here they are able to outcompete other algae because of their ability to fix nitrogen in water that has little available nitrogen. Cyanobacteria that do not fix nitrogen have reduced growth, and therefore reduced buoyancy, and sink in the water column. Once established, a bloom of buoyant, nitrogen-fixing, cyanobacteria tends to be self-perpetuating in that increased mass of the bloom maintains the reduced light and CO2 levels required for maximum buoyancy.
Structures other than gas vesicles can cause significant variation in cell density, and therefore buoyancy (Konopka et al., 1987). Polyphosphate granules may have a density of 2 g cm 3 or greater, and glycogen (which is accumulated under high light intensities) has a density of about 1.5 cm 3. Both have a higher density than water (1 g cm 3) and can cause cells to sink (Booker and Walsby, 1981; McCausland et al., 2005).
Pigments and photosynthesis
The major components of the photosynthetic light-harvesting system of the cyanobacteria are chlorophyll a in the thylakoid membrane, and the phycobiliproteins, which are water-soluble chromoproteins assembled into macromolecular aggregates (phycobilisomes) attached to the outer surface of the thylakoid membranes. Some cyanobacteria contain chlorophyll b and the cyanobacterium Acaryochloris marina (Fig. 2.20) contains chlorophyll d. At one time those cyanobacteria containing chlorophyll b (Prochlorococcus (Fig. 2.20),
Prochlorothrix, Prochloron) were thought to be a distinct evolutionary group and they were placed in the Prochlorophyta. Evolutionary trees based on nucleic acid sequencing have shown that chlorophyll b arose a number of times and that these cyanobacteria are spread throughout the group
(Palenik and Haselkorn, 1992; Urback et al., 1992). In contrast, Acaryochloris marina (Fig. 2.20), the only cyanobacterium known to have chlorophyll d, appears to be only distantly related to other cyanobacteria (Miller et al., 2005; Miyashita et al., 2003). The absorption spectrum of chlorophyll d (Fig. 1.19) is shifted toward far-red wavelengths and A. marina exists in environments where there is an abundance of these wavelengths of light (e.g., under red algae).
The carotenoids of the cyanobacteria differ from those of the eukaryotic algae in having echineone (4-keto- -carotene) and myxoxanthophyll, which eukaryotic algae do not have; in lacking lutein, the major xanthophyll of chloroplasts; and in having much higher proportions of -carotene than are found in eukaryotic algae (Goodwin, 1974).
The Cyanophyceae have four phycobiliproteins: C-phycocyanin (absorption maximum at a wavelength [ ] of 620 nm), allophycocyanin ( max at 650 nm), C-phycoerythrin ( max at 565 nm),
and phycoerythrocyanin ( max at 568 nm). All cyanobacteria contain the first two, whereas
C-phycoerythrin and phycoerythrocyanin occur only in some species. The phycobiliproteins of the cyanobacteria change in concentration in response to light quality and growth conditions. Cyanobacteria that produce the red phycoerythrin and the blue phycocyanin in white light, suppress phycoerythrin synthesis in red light and phycocyanin synthesis in green light (comple- mentary chromatic adaptation; see Tandeau de Marsac, 1977).
In the evolution of the cyanobacteria, the thylakoids probably originated by invaginations of the plasmalemma; some cyanobacteria today have thylakoids that are continuous with the plasmalemma. An example of the primitive condition may be Gloeobacter violaceus (Fig. 2.19(e)), a unicellular cyanobacteria that lacks thylakoids but has chlorophyll a, carotenoids, and phycobiliproteins. In this alga the pigments, and presumably photosynthesis, are associated with the plasmalemma (Rippka et al., 1974).
Many cyanobacteria have the ability to photosynthesize under aerobic or anaerobic conditions. Under aerobic conditions, electrons for photosystem I are derived from photosystem II. Under
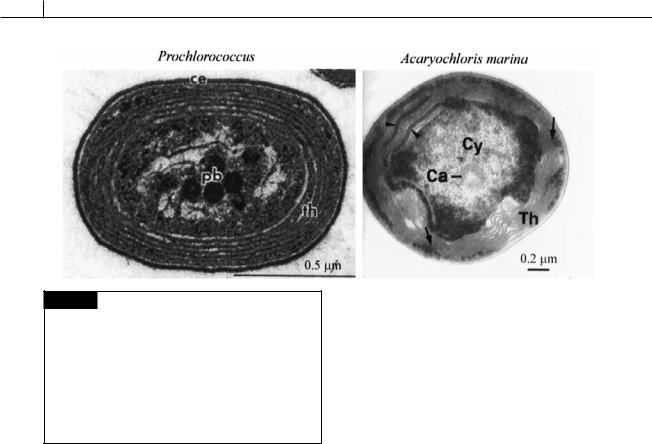
44 THE PROKARYOTIC ALGAE
Fig. 2.20 Transmission electron micrographs of a section of Prochlorococcus, a chlorophyll b containing cyanobacterium, and of Acaryochloris marina, a chlorophyll d containing cyanobacterium. Prochlorococcus is the smallest known photosynthetic organism. (Ca) Carboxysome (polyhedral body); (Cy) cytoplasm; (pb) polyhedral body; (th) thylakoids. (Arrow) channel-like structures perforating thlyakoids. (Arrowheads) areas of accumulation of phycobiliproteins. (Prochlorococcus micrograph from Partensky, Hess and Vaulot, 1999; Acaryochloris micrograph is from Marquardt et al., 2000.)
anaerobic conditions, in the presence of sulfur, electrons are derived by the reduction of sulfur:
light
CO2 2H2S → (CH2O) H2O S chlorophyll sugar
These cyanobacteria are facultative phototrophic anaerobes and fill an important ecological niche in aquatic systems (Padan, 1979). Eukaryotic algae are restricted to photoaerobic habitats, whereas photosynthetic bacteria are restricted to photoanaerobic habitats. In habitats that fluctuate between the above conditions, cyanobacteria with facultative anaerobic photosynthesis have a clear selective advantage. An example of this is the Solar Lake, Elat, Israel, where in the winter high levels of sulfide are found in the anaerobic bottom layers of water of the thermally stratified lake. Oscillatoria limnetica (Fig. 2.19(b)) occurs in these highly anaerobic bottom layers, where sulfide functions as an electron donor for photosynthesis. In the spring, the lake overturns, with all of the water becoming aerobic, Oscillatoria limnetica activates aerobic
photosystem II (Fig. 2.28) and carries out photosynthesis aerobically. Thus O. limnetica, by utilizing combined anoxygenic and oxygenic photosynthesis, is the dominant phototroph of the Solar Lake, with its fluctuating photoaerobic and photoanaerobic conditions. The interlinking position of the cyanobacteria in the phototropic world is compatible with the fact that they are among the oldest organisms, dating back to the Precambrian Period. Significantly, two of the sulfide-rich ecosystems containing high numbers of cyanobacteria – that is, hot sulfur springs and the marine littoral sediments – may represent old ecosystems that may predate the oxidized biosphere.
Photosynthesis in many cyanobacteria is stimulated by lowered oxygen concentration, the oxygen competing with carbon dioxide for the enzyme ribulose-1, 5-bisphosphate carboxylase/ oxygenase (Fig. 2.21) (Stewart and Pearson, 1970; Weller et al., 1975). This phenomenon probably reflects an adaptation to the absence of free oxygen in the atmosphere of Precambrian times when the cyanobacteria first evolved. After the evolution of the oxygen-evolving cyanobacteria, the oxygen in the atmosphere gradually built up, creating a protective ozone (O3) layer in the atmosphere at the same time. The ozone layer removed most of the harmful ultraviolet radiation from the sun and allowed the evolution of more radiationsensitive organisms. The cyanobacteria are relatively insensitive to radiation, having a system that repairs radiation damage (Bhattacharjee, 1977).