
книги / Структурно-механические свойства эластомерных композитных материалов
..pdfМинистерство образования и науки Российской Федерации
Федеральное государственное бюджетное образовательное учреждение высшего образования
«Пермский национальный исследовательский политехнический университет»
А.С. Ермилов, Э.М. Нуруллаев
СТРУКТУРНО-МЕХАНИЧЕСКИЕ СВОЙСТВА ЭЛАСТОМЕРНЫХ КОМПОЗИТНЫХ МАТЕРИАЛОВ Избранные статьи
Издательство Пермского национального исследовательского
политехнического университета
2018
УДК 678.0232+678.027 Е72
Ermilov, A.S.
Structural and mechanical properties of elastomeric composite materials. Selected works / A.S. Ermilov, E.M. Nurullaev. – Perm: Publisher of the Perm National Research Polytechnic University, 2018. – 223 s.
ISBN 978-5-398-01978-0
The book of selected scientific works contains theoretical and experimental foundations for the construction of elastomeric composite materials for use in units and details of transport equipment designs with a wide temperature range of operation, including the regions of the Far North and the Arctic of Russia.
For the first time, the dependence of the energy (work) of mechanical failure under uniaxial tension conditions on the main structural parameters of filled plasticized three-dimensionally cross-linked elastomers based on high molecular weight copolymers such as polydiwinylisoprene versus composites based on low molecular weight rubbers with terminal functional groups is described for the first time.
The book is recommended for vehicle designers, including aviation and automotive engineering. As a textbook selected works are useful for young engineers, graduate students, undergraduates and students of relevant specialties.
Ермилов, А.С.
Е72 Структурно-механические свойства эластомерных композитных материалов : Избранные статьи / А.С. Ермилов, Э.М. Нуруллаев. – Пермь : Изд-во Перм. нац. исслед. политехн. ун-та, 2018. – 223 с.
ISBN 978-5-398-01978-0
Представлены теоретические и экспериментальные основы построения эластомерных композитных материалов для использования в узлах и деталях конструкций транспортной техники с широким температурным диапазоном эксплуатации, включая районы Крайнего Севера и Арктики России.
Впервые описана зависимость энергии (работы) механического разрушения в условиях одноосного растяжения от основных структурных параметров наполненных пластифицированных трёхмерно сшитых эластомеров на основе высокомолекулярных сополимеров типа полидивинилизопрена в сравнении с композитами на основе низкомолекулярных каучуков с концевыми функциональными группами.
Рекомендуется для конструкторов транспортных средств, включая авиационную и автомобильную технику, а также издание будет полезным молодым инженерам, аспирантам, магистрантам и студентам технических вузов.
УДК 678.0232+678.027
ISBN 978-5-398-01978-0 |
© ПНИПУ, 2018 |
PREFACE the AUTHORS
Modern scientific and technological progress in Russia is characterized by intensive development of elastomer composite materials for use in sites and detail designs of transport technology with wide temperature range exploitation, including areas of the far North and the Arctic. Decision Engineering Task "How to make a polymer composite material with required mechanical characteristics?" can only be satisfied when you post the main structural parameters of compositions on the Atomic-molecular level and the geometry of the particles dispersion filler.
A collection of the most important scientific articles "Structuralmechanical properties of elastomer composite materials" published in reputable international journals dedicated to the development of physicomathematical theory in thisarea. First described by energy dependence of mechanical destruction in conditions of uniaxial stretching from the main structural parameters of plasticized filled three dimensional cross-linked elastomer.
Ermilov A.S., Nurullaev E.M.
ПРЕДИСЛОВИЕ АВТОРОВ
Современный научно-технический прогресс в России характеризуется интенсивной разработкой эластомерных композитных материалов для использования в узлах и деталях конструкций транспортной техники с широким температурным диапазоном эксплуатации, включая районы Крайнего Севера и Арктики. Решение инженерной задачи «Как сделать полимерный композитный материал с требуемыми механическими характеристиками?» можно осуществить только при учёте основных структурных параметров композиций на атомно-молекулярном уровне и геометрии частиц дисперсного наполнителя.
Сборник важнейших научных статей «Структурно-механические свойства эластомерных композитных материалов», опубликованных авторами в авторитетных международных журналах, посвящён развитию физико-математической теории в указанной области. Впервые описана зависимость энергии механического разрушения в условиях одноосного растяжения от основных структурных параметров наполненных пластифицированных трёхмерно сшитых эластомеров на основе высокомолеку-
3
лярных и низкомолекулярных каучуков сополимерного типа. Представлены соответствующие математические уравнения для начального модуля вязкоупругости, зависимости условного напряжения от относительного удлинения и энергии механического разрушения. Построены огибающие разрушения и значений энергий разрыва при различных температурах испытаний.
Ермилов А.С., Нуруллаев Э.М.
4
Mechanics of Composite Materials, 2012, Vol. 48, No. 3
MECHANICAL PROPERTIES OF ELASTOMERS FILLED
WITH SOLID PARTICLES
A. S. Ermilov and E. M. Nurullaev
Keywords: filled elastomer, mechanics, influence of structure.
A physical-mechanical description of the mechanical characteristics of a three-dimensional cross-linked plasticized elastomer filled with solid particles is presented. The basic structural parameters of the composition and the temper- ature-rate equivalence in the uniaxial tension of specimens are taken into account. Examples of solution of direct and inverse prediction problems are given.
INTRODUCTION
Polymer composite materials with an elastomeric matrix filled with solid particles are widely used in parts and units of various structures in the automotive, aircraft, and shipbuilding industries. Abrasive compositions containing corundum (alumina) make it possible to perform high-quality grinding in mechanical engineering. Elastomers filled with silica of different types and fractional compositions are employed in the building industry, including roof coverings of buildings and floorings in sports constructions.
Mechanical characteristics of the materials mentioned considerably affect the service life of particular parts or units of structures. In this case, the most important factors are the molecular structure of the polymeric basis, the type and degree of its plasticization, and the volume degree of effective filling, which depends on the shape and fractional composition of dispersed filler particles and on the physical and chemical interaction at the elastomer–filler interface.
The phenomenological Mooney–Rivlin equation, which is presented in the review [1] and is based on the elastic potential of a deformed interstitial chain in a polymer network, formally rather well describes the relation between the conditional stress (tensile force related to the initial cross section) and elongation of a specimen. However, this equation does not reflect explicitly the effect of basic physical and chemical parameters of the elastomeric composition on the above-mentioned relation. In this connection, the purpose of the present study is the development of physical and chemical foundations
5
of the mechanical behavior of an elastomer filled with solid particles, with the Mooney–Rivlin equation adopted as a functional core. To achieve this goal, we suggest
-a normalized temperature-rate relationship for the “physical” (intermolecular) component of transverse bonds in a polymeric binder;
-an analytical relation between the conditional stress and elongation which takes into account the strengthening of a plasticized elastomeric matrix by particles of solid constituents.
PHYSICAL AND MATHEMATICAL DESCRIPTION
Let us consider the structural-mechanical behavior of a threedimensionally cross-linked plasticized elastomer filled with solid particles in uniaxial tension. The elastic potential of a spatial polymer network, on the basis of which Mooney and Rivlin deduced their equation, has the fo
U C1(I1 3) C2 (I2 3), |
(1) |
where U is the elastic potential of an interstitial molecular chain, equal to the internal energy accumulated at the expense of the work performed in tension of a specimen; I1, I2 and are invariants of the second-rank elongation tensor
when the relative elongations and are xx 1, yy 2, zz 3) directed
along the axes of a Cartesian system of coordinates; C1 and C2 are halves of the parameters entering into the Mooney–Rivlin equation;
I1 12 22 32; I2 12 22 22 32 32 12, I3 12 22 32 1.
The relative elongation αi is connected with the strain εi by the relations
2 |
1 2 ; |
2 |
1 2 |
; |
2 |
1 2 |
3 |
1 |
1 |
2 |
2 |
|
3 |
|
if the Poisson ratio ν is equal to 0.5.
The parameters 2C1 and 2C2 allowed Mooney and Rivlin to write their equation in the form
(2C 2C 1)( 2), |
(2) |
|
1 |
2 |
|
where σ is the conditional stress (tensile force related to the initial cross section).
Up to now, the parameters 2С1 and 2С2 have been associated with the chemical and “physical” (intermolecular) components of transverse bonds in
6
the polymeric basis of a binder. Really, from Eq. (2) formally follows the expression
2C ( 2) 2C 1 |
( 2), |
(3) |
|
1 |
2 |
|
|
in which the first term corresponds to the equilibrium one: T Tg 2000 se of
elasticity theory of cross-linked rubbers (resins) at a test temperature close to the equilibrium one: (Tg is the temperature of structural glass transition of a polymer). Then, as follows from [1],
2C ( 2 ) |
ch |
kN T ( 2) |
RT ( 2 ). |
(4) |
1 |
A |
ch |
|
Here, νch is the molar concentration of chemical transverse bonds in the cross-linked polymer; k is the Boltzmann constant; NA is the Avogadro number; R is the gas constant per mole; (1 /100) is the relative elongation
as a function of strain.
To verify the applicability of Mooney–Rivlin equation (2) to the case of a filled elastomer and the effect of the second term in Eq. (3), we put to use its linear form
/ ( 2) 2C 2C 1. |
(5) |
|
1 |
2 |
|
Experimental results (reconstructed tension diagrams) for an elastomeric composition filled with silica were presented in Fig. 1. The physical and chemical features of the composition are as follows. The polymeric basis is formed by copolymerized low-molecular rubbers with epoxy (PDI-3B) and carboxyl SKD-KTR) end groups, transversely cross-linked by an EET-1 threefunctional epoxy resin. The filler is a mixture of two fractions of silica: a natural macro crystalline quartz (500-1500 μm) and a highly dispersed pyrogenetic amorphous quartz of trademark “Aerosil-380” (35-40 nm) in the ratio 80 : 20. The volume fraction of filler was 0.712.
It is seen that, also in the case of the type of filled elastomer considered, the test data are rather well described by the Mooney–Rivlin equation. This allows us, with account of formula (5), to determine the parameters 2С1 and 2С2 graphically. The quantity 2С2 in Eq. (5), which is related to the viscous (relaxation) component of the initial viscoelastic modulus ( E d / d at α =1), tends to zero (intermolecular bonds fail) with rising temperature and decreasing strain rate (see Fig. 1). In addition, the quantity 2С1, which is con-
7
nected with the elastic component of the initial viscoelastic modulus, remains practically constant.
The coefficient of the inversible temperature dependence of change in 2С2 for a filled elastomer can be written as aT (2C1 2C2 ) / 2C1 in the tem-
perature range from Tg to T∞, approximately equal to 200K for the majority of 3D cross-linked polymers. This fact leads to a 30-fold change in the total number of chemical (constant) and “physical” (inversibly varying) bonds.
Figure 2 shows experimental data of a normalized relation between the initial viscoelastic modulus Е and the reduced temperature (Т – Тg) for different elastomers filled with silica. Taking into account the character of the curve drawn through the experimental points (see the figure), after some mathematical processing, we arrived at following empirical formula:
ET |
ET g |
|
|
|
3 |
(T Tg ) |
2 |
|
|
|
|
exp |
0,225 |
10 |
|
|
, |
(6) |
|
ET |
ET |
|
|||||||
g |
|
|
|
|
|
|
|
|
|
The experiments were carried out on elastomers of different chemical nature, filled with silica of the fractional composition mentioned. As polymeric binders, we used polyesterurethane, polydiene epoxy urethane, and polybutadiene, nonplasticized and plasticized by dibutyl phosphate and dioctyl sebacate, and transversely cross-linked by the corresponding curing systems. The volume fraction of filler was 0.712-0.785. Expression (6) is equivalent to the parameter 2С2 In view of dependence (6), the Mooney–Rivlin equation is refined regarding the influence of the “physical” (intermolecular interaction) component of transverse bonds in the polymeric binder:
ch r1/3RT 1 29exp 0,225 10 3 (T Tg )2 1 a 1 ( 2 ). (7)
Here, for a plasticized elastomer, r (1 sw ) 1is the volume fraction of the polymeric base of binder; ϕsw is the volume fraction of softener in the elastomer; Т is the test temperature, a 1 is the shift factor taking into account
the effect of strain rate (equal to unity for the standard rate of uniaxial tension).
8
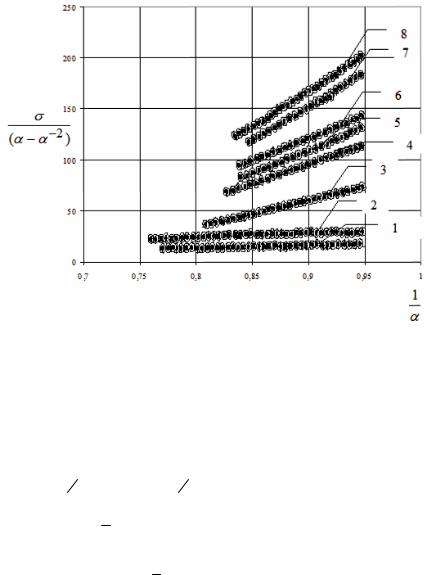
Fig. 1. Relationships / ( 2 ) f ( 1 ) (reconstructed tension diagrams)
for an elastomeric composition filled with silica at Т = 323 (1), 293 (2, 4, 6), 273 (3), 253 (5), 233 (7), and 223 K (8) and strain rates 1,2·10-3 s-1; –3 (1-3, 5, 7, 8), 4.6·10–4 (4), and 4.6·100 s–1 (6)
Thus, the effective concentration of transverse bonds, equal to the concentration of temperature-dependent chemical and physical bonds, is
eff |
13 |
13 |
1 29exp |
|
0,225 |
10 |
3 |
T Tg |
2 |
ch r |
1 ph ch r |
|
|
|
Here, ch / Мs where ρ is the density of polymer, and Mc is the average interstitial molecular weight of polymer basis of the elastomeric binder. For calculating the quantity Мs , we can construct the corresponding molecu-
lar graph reflecting the formation of a network (spatially cross-linked) elastomer on the basis of low-molecular rubbers (SKD-KTR and PDI-3B) with end functional groups, some of which chemically react with the antipode groups of the cross-linking three-functional agent – EET-1 epoxy resin. Figure 3 shows a three-dimensional molecular graph and reflects the “participation” of the cross-linking agent – EET-1 three-functional epoxy resin.
9
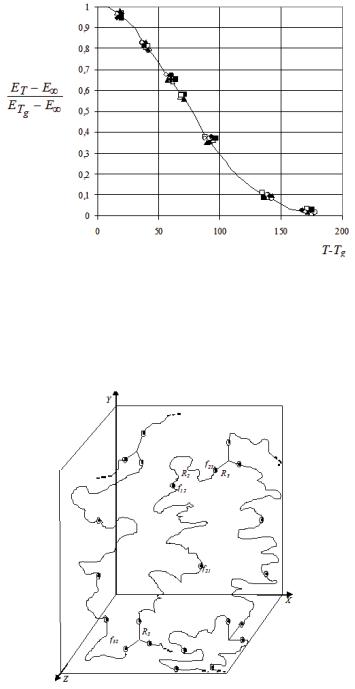
Fig. 2. Normalized relationship between the current viscoelastic modulus ET E ETg
and the temperature Т–Тg for various elastomers filled with silica: ▲ – polysulfide, ♦ – polyesterurethane, ○ – polyisoprene divinyl, ◊ – polybutadiene,
□ – polybutylisoprene, and ■ – polydiene epoxy urethane
Fig. 3. Graphic simulation of a three-dimensional network formed by two bifunctional rubbers R1 and R2 and resins R3 with functional groups: f1, f2 – epoxy and f3 – carboxyl
10