
книги / Структурно-механические свойства эластомерных композитных материалов
..pdf
filled with silica (SiO2) of varying degree of fineness: 1 – 1µm; 2 – 5µm; 3 – 15µm; 4 – 240µm; 5 – 600µm; 6 – a mixture of 2 or 3 fractions taken in the optimal ratio in terms of the minimum porosity for increasing the maximum volume filling of the composition ( m ).
Correlation analysis shows that the most probable value of the combined parameter K (Р =0.95) at the pole of the concurrent lines in the given coordinates equals to 1.25, whereasC1 2K 2.5 . The presence of the "pole" is in-
dicative of the invariant nature of the concentration dependence. It is interesting to note that the formulas (1) and (5) can be transformed into the wellknown theoretical Einstein’s formula for viscosity of dilute suspensions (Einstein, 1920): f o (1 2.5 ) at m 1.
Fig. 1. The dependence ( Rf 1) 1 f ( ) 1 for the compositions based on low-
molecular-weight polybutadiene SKD-KTR grade rubber with terminal carboxyl
groups filled with silica (SiO2) of varying degree of fineness: – 1µm;
– 5µm;
– 15µm;
– 240µm;
– 600µm;
,
,
,
– a mixture of 2 or 3 fractions taken in the optimal ratio in terms of the minimum porosity for increasing the maximum
volume filling of the composition ( m )
61
According to Brouwers H. J. H (2010), a theoretical justification of the dependence of the relative viscosity of concentrated rubber suspensions and the viscoelastic modulus of 3D cross-linked elastomers filled with solid particles has been provided for the first time. We have also detected the pole which validates the invariant value of the constant of the developed equation.
Thus, the theoretical dependence (5) corresponds to the formula (1), which (alongside with our experimental data and the results of Chong et al. (1971) and Fedors (1979)) is recommended for use in calculating the coefficient of reinforced rubber suspensions and the viscoelastic modulus of 3D cross-linked elastomers filled with solid particles.
EXPERIMENTAL STUDY
Ermilov et al. (2011) verified the formula (1) in terms of enhancement of the initial viscoelastic modulus of 3D cross-linked elastomers for developing a frost-resistant waterproofing rolled material for asphalt coatings.
Various low-molecular-weight rubbers (polyethylene butyl formal, polyester urethane, polybutadiene, polydiene epoxy urethane) with terminal functional groups ( SH , OH , COOH , CH (O)CH2 ) and high-molecular-
weight unsaturated rubbers (polyisoprene butyl, polyisoprene divinyl) threedimensionally crossed-linked by means of the functional groups, including double bonds (-CH = CH-), were used as polymer binders.
The cross-linking agents were compounds having the antipodal functional groups capable of forming the necessary chemical bonds. For example, the reaction between the isocyanate group and the hydroxyl one results in the urethane group which links two molecules of the polymer. Some high- molecular-weight and low-molecular-weight rubbers contained a plasticizer as a component of the polymer binder.
It should be noted that the formation of the elastic and viscous components of the initial modulus at a uniaxial stretching (elongation) of the specimens was as follows. The concentration of the transverse chemical bonds in the 3D cross-linked polymer binder determined the elastic component of the modulus, whereas the degree of polarity of molecular groups of the binder components (including plasticizer) determined the viscous component of the modulus. In contrast to the elastic component, the viscous component of the modulus is in inverse proportion to temperature and strain rate due to the nature of intermolecular interactions (Nielsen, 1978). The dispersed filler was
62
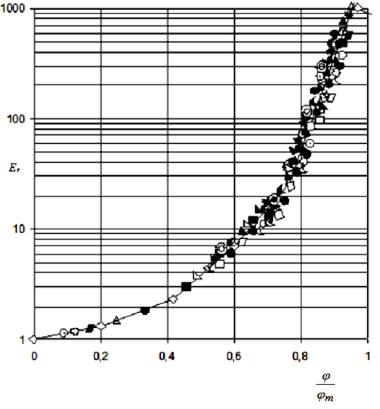
a three-fraction mixture of silica (river quartz sand) with the optimal size grading 600 : 240 : 15 µm = 50: 30: 20. The fraction of 15 µm (as well as the other fractions 1 µm and 5 µm) was obtained by grinding quartz sand in the vacuum
grinder. Fig. 2 shows the calculated dependence Er Ef / Eo f ( / m ) and
the experimental data for filled elastomers based on these rubbers. It is evident that the result of the experimental verification of the theoretical approach is in agreement with the data of Fedors (1979) and Chong et al. (1971) both in terms of the dynamic viscosity coefficient (Fig. 1) and the relative initial viscoelastic modulus of different polymer compositions (Fig. 2).
Fig. 2 shows the calculated dependence Er E f / Eo f ( / m ) and the experimental
data for filled elastomers based on these rubbers: 1 – (,
,
,
) polyethylene butyl formal 2 – (
,
,
) polyester urethane, 3 – (
) polybutadiene, 4 – (
,
,
) polyisoprene butyl, 5 – (
,
,
) polyisoprene divinyl, 6 – (
,
,
) polydiene epoxy urethane filled with polydisperse silica
63

ENGINEERING APPLICATIONS
The formula of reinforcement of rubbers and 3D cross-linked elastomers
(1) allows, alongside with the calculation methods proposed by Ermilov and Fedoseev (2004), to determine the value of the maximum filling of the polymer binder with separate filler fractions or polydisperse fractions based on them and to minimize the number of the experiments. Indeed, the transformation of the equation (1) in relation to m results in the following formula:
m |
1.25 ( |
f / o 1) |
, |
(7) |
|
f o |
|||||
|
|
|
which allows to estimate the value of m by a viscometric method. This pro-
vides a more accurate estimation of the maximum filling compared to determination of the packing density of the particles in bulk since it "automatically" takes into account the intermolecular interaction at the filler – binder interface. Therefore, the most appropriate binder is low-molecular-weight polybutadiene SKD-KTR grade rubber with terminal carboxyl groups of medium polarity in terms of the immobilizing effect of the dispersed filler on the molecular mobility of the binder. The coefficients of dynamic viscosity of the binders and filled compositions based on them were determined using Heppler consistometer at one of the moderate values of φ and 3-5 parallel measurements. The levels of the gradients of shear rate and experimental temperatures were selected in compliance with practical needs and interests of the particular branches of science and industry.
Table 1 presents the results of viscosimetric determination of the maximum filling of polybutadiene rubber with various fractions of silica used in the experiments.
Table 1. The maximum filling of low-molecular-weight polybutadiene SKD-KTR grade rubber with various fractions of silica
Weight-average particle size of silica |
|
|
|
|
|
fractions in the mixture with SKD-KTR |
1 |
5 |
15 |
240 |
600 |
rubber, µm |
|
|
|
|
|
The maximum filling of polybutadiene |
|
|
|
|
|
SKD-KTR grade rubber with various |
0.530 |
0.547 |
0.614 |
0.699 |
0.640 |
fractions of silica, m |
|
|
|
|
|
64
It is clear that with increase in the degree of mechanical grinding (fractions 1, 5, 15 µm) the value of m decreases significantly, apparently due to
the more angular particles. The fractions 240 µm and 600 µm screened out from river quartz sand feature particles of more rounded shapes, which are more favorable in shear flows of the compositions.
Nielsen (1978) studied the physical properties and the chemical structure of the polymeric base of the binder and plasticizer and concluded that they affect the intermolecular interaction at the filler-binder interface. As a result of the immobilizing effect, the mobility of the molecules at the filler-binder interface decreases, which reduces the value of the maximum volume filling, all other conditions being equal. Therefore, less polar molecules of the binder components show higher values of m .
Table 2 presents the immobilizing effect of the physical and chemical properties of the polymeric binder on its maximum filling with a three-fraction mixture of silica with the optimal size grading 600: 240: 15 µm = 50: 30: 20.
Table 2. The influence of physicochemical features of the polymer binder on its maximum filling with polydisperse silica
Polymer binder filled with a three-fraction mixture |
Maximum |
of silica |
volume filling, m |
P-9А grade polyester |
0.770 |
NVTS-2 grade polyethylene butyl formal |
0.810 |
Polydiene epoxy urethane PDI-3B grade rubber plasti- |
0.830 |
cized by dioctyl sebacate (30%) |
|
BK grade polyisoprene butyl plasticized by transformer |
0.910 |
oil (80%) |
|
SKID-L grade polyisoprene divinyl plasticized by trans- |
0.940 |
former oil (70%) |
|
CONCLUSIONS |
|
1. For the first time, we have presented a theoretical justification and an experimental validation of the formula (1) which invariantly describes the concentration dependence of reinforcement of rubbers and 3D cross-linked elastomers. We have also first located the "pole" of the experimental data of the equation (5) in the coordinates of the linear form (6) that substantiates the value of the constant (К = 1.25).
65
2.On the basis of the obtained dependence we have developed a viscometric method of determining the maximum volume filling of polymer binders (7) with solid particles of varying fractional composition.
The use of computational methods for the determination of the maximum filling of the polymer binder with polydisperse mixtures as the initial viscometric values of the maximum filling with separate fractions significantly increased accuracy.
3.The experiments showed that less polar molecules of the binder components provide the value of the maximum filling of the elastomeric binder with three-fractional mixtures of silica at the level of 0.95, all other conditions being equal. This reduces the coefficient of dynamic viscosity of the filled elastomer and its initial viscoelastic modulus in a 3D cross-linked state.
4.The results of the studies are recommended for use in the accelerated development and optimization of new formulations of polymeric composite materials using mathematical methods, for example, the simplex-lattice design for experiments.
REFERENCES
1.Chong J.S., Christiansen E.B., Baer A.D. Rheological Properties of Concentration Suspensions // J. Appl. Polym. Sci. – 1971. – Vol. 15. – P. 2007-2024.
2.Eilers H. Die Zahigkeit eine konzentrieren Suspensionen // Kolloid. Z. 1941. – Band 97. – P. 313-317. Einstein A. Viscosity of the Dilution Suspensions // Kolloid. Zeitschrift. – 1920. – Vol. 27. – №. 55. – P. 137-139.
3.Ermilov A.S., Nurullaev E.M. Mechanical Properties of Elastomers Filled with Solid Particles // Mechanics of Composite Materials. – 2012. – Vol. 48. – № 3. – Pp. 243-252.
4.Ermilov A.S., Fedoseev A.M. Combinatorial-Multiplicative Method of Calculating the Limiting Filling of Composites with Solid Dispersed Components // Journal of Applied Chemistry. – 2004. – T. 77. – Issue. 7. – P. 12181220.
5.Ermilov A.S., Nurullaev E.M., Alikin V.N. Waterproof Frost-resistant Road Asphalt Coating. Patent number 2473581, Russian Federation. Date of filing: 31. 05. 2011.
6.Fedors R. Effect of Filler on the Mechanical Behaviour of Elastomers. Relationships between the Small Strain Modulus and the Type and Concentration of Filler // Polymer. – 1979. – Vol. 20. – № 3. – P. 324-328.
66
7.Moony M. Rheology of Concentration Suspensions // J. Coll. Sci. – 1951. Vol. 6. – P. 162-156.
8.Nielsen L.E. Mechanical Properties of Polymer and Polymer Composition. –M.: Chemistry, 1978. P. 310.
9.Rutgers J.R. Relative Viscosity and Concentration // Rheologica Acta. – 1962. – Vol. 2. – № 4. – P. 305-348.
Mechanics of Composite Materials, 2013, Vol. 49, No. 3
OPTIMIZATION OF FRACTIONAL COMPOSITION OF THE FILLER
OF ELASTOMER COMPOSITES
A. S. Ermilov and E. M. Nurullaev
A method is developed for calculating the optimum fractional composition of an initial disperse filler of polymer compositions that significantly affects the initial viscoelastic modulus and the fracture energy of threedimensionally cross-linked elastomer composites. For a particular composite, the practical efficiency and usefulness of the proposed method using the triangular Gibbs diagram of “composition–property” have been verified experimentally.
Keywords: elastomer, optimization, fractional composition, filler
INTRODUCTION
The fractional composition of particles of solid components is of principal importance for the formation of mechanical properties of the filled elastomers widely used in rubber products in mechanical engineering, ship and aircraft production, and building industry. The worldwide success of, for example, automobile tires is explained by the polymer base of rubber, namely a three-dimensionally cross-linked rubber being in a highly elastic state over a wide range of operating temperatures. An actual problem today is the creation of rolled frostand hydroresistant asphalt coverings of highways located in zones with a sharply continental climate. In [1], the relation between the mechanical properties of an elastomer filled with solid particles and basic parameters of the composition was already investigated both theoretically and experimentally. The development of the method for calculating the optimum
67
fractional composition of particles of an initial disperse filler of polymer composite materials is a particularly topical problem. This is connected with reduced prices of the respective materials due to minimization of the volume content of the polymer binder as the more expensive component compared with, for example, silica (quartz sand).
As a criterion of the method developed, we chose the initial viscoelastic modulus of three-dimensionally cross-linked low-molecular rubbers with end functional groups, filled with polyfractional solid particles. The application of the method is illustrated by an engineering example of optimization of a fractional composition of filler particles ensuring the minimum value of relative initial viscoelastic modulus of the filled elastomer. We investigate the effect of density of a package of the initial filler particles on the energy (work) of mechanical fracture of an elastomer composite in uniaxial tension in the form of “enveloping” fracture curves [2]. The case of unbroken solidity of the composite up to its failure is considered. Exactly this case is of practical interest in solving the problem of multiply increasing the service life of highways subjected to the destructive effect of repeated water–ice phase transitions.
One of the basic structural (formulation) parameters of polymer composites is the effective volumetric degree of filling jjm, where j is the volume fraction of solid particles and jm is the limiting volumetric degree of filling, depending on the shape of particles and their size distribution and on the physical and chemical interactions at the filler–binder interface. The quantity jm, which is connected with density of the chaotic package of particles of the initial filler, can be determined by viscometry [3] or calculated by the combinato- rial-multiplicative method [4].
For the relative initial viscoelastic modulus Еr , which is determined by jjm, the invariant relation [3]
Er |
Ef |
|
|
/ |
m |
2 |
(1) |
|
|
1 |
1,25 |
|
|
, |
|||
Eo |
|
|
||||||
|
|
|
1 / m |
|
|
is valid, where the subscripts “f” and “o” refer to the filled and free states of the polymeric binder of the composite.
The structural-mechanical relation between the conditional (related to the initial cross section of a specimen) stress σ and the stretch ratio ,1 /100, where ε is the strain, of filled elastomers, has the form [1]
68
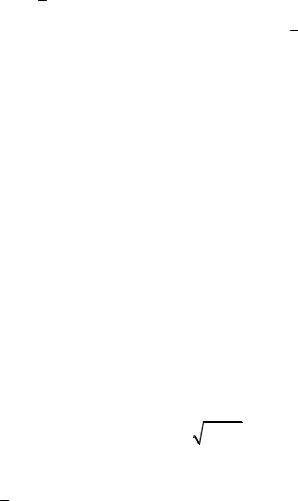
( ) ch r1/3RT 1 29exp 0,225 10 3 (T Tg )2 1a 1
|
|
/ m |
|
2 |
(2) |
|
|
( 2 ), |
|||
1 |
1,25 |
|
|
||
|
|
||||
|
|
1 / m |
|
|
Here, ch / Мs is the concentration of transverse chemical bonds in the
polymeric base of binder, ρ is the density of polymer, Мs is the average inter-
stitial molecular weight; φr is the volume fraction of polymer in the plasticized binder; R is the gas constant per mole; T∞ is the equilibrium temperature at which the concentration of intermolecular (“physical”) bonds νph in the polymeric binder is negligibly small; Т is the testing temperature of the composite material; Tg is the structural glass-transition temperature of the polymer binder; a is the coefficient of high-speed displacement; is the relative tension
rate of the specimen. As follows from Eq. (2), the initial viscoelastic modulus of the filled elastomer is
d |
|
|
|
1/3 |
|
|
|
|
|
|
10 |
3 |
|
2 1 |
|
|
|
|
|
|
|
|
|
|
|||||||
Ef d |
|
1 |
3 ch |
|
RT |
1 29exp 0,225 |
|
T Tg |
a |
|
|||||
|
|
|
|
||||||||||||
|
|
|
|
|
|
|
|
|
/ m |
2 |
|
|
|
|
(3) |
|
|
|
|
|
1 |
1,25 |
|
|
. |
|
|
|
|
|
|
|
|
|
|
|
|
|
|
|
|
|
|
|
|||
|
|
|
|
|
|
|
1 |
/ m |
|
|
|
|
|
The fracture envelopes in the coordinates "log b log b "− were constructed by using the relation between the breaking stress and strains of the filled ( bf , ob ) and free ( ob , ob ) elastomers
bf |
ob (1 3 / m ), |
(4) |
where the invariant relationship between εob and the effective concentration of transverse chemical and intermolecular (physical) bonds [νeff = νch + νph, where
νch = / Mc ] was found experimentally in [1] for different 3D cross-linked
elastomers. The energy of mechanical fracture of the filled elastomer was determined as the area under the tensile diagram of a specimen:
b |
|
Ab ( )d , |
(5) |
1 |
|
where the function σα is expressed by Eq. (2).
69
The purpose of the present investigation is a theoretical development and an engineering application of the method (algorithm) to calculating the optimum fractional composition of particles of an initial disperse filler as applied to polymer composite materials, in particular, elastomeric composites. We employed the division of the theory of investigation of operations – the nonlinear mathematical programming [5-7] – and modern practical methods of optimization [8].
THEORETICAL PART
The problem of optimization of the fractional composition of solid disperse components of a polymer composite (for assigned mean-weight sizes of particles of fractions) for an optimization criterion chosen, e.g., for the relative initial viscoelastic modulus [with account of combination of formulas (1) and (3): [Er = Ef/E0] can be formulated as the following statement of nonlinear programming [9]:
|
|
m |
|
|
|
|
|
|
|
|
at Er min |
|
|
|
m j |
||||||
|
|
,q,d |
max |
opt |
|
|
|
|
|
|
|
|
|||||||||
|
|
|
|
|
j1 |
j 2 |
j3 |
jmj |
j |
||||||||||||
|
|
|
|
|
|
|
|
j |
|
|
|
|
|
|
|
|
1 |
||||
|
0 minjv |
jv maxjv |
1, |
v 1, |
2, |
3, ..., mj |
at j In; |
||||||||||||||
|
|
|
|
|
|
|
opt |
|
xoptj |
/ j |
|
|
|
|
|
|
|
||||
|
|
|
|
|
|
j |
|
|
|
, |
|
|
|
|
|
||||||
|
|
|
|
|
|
|
xoptj |
/ j |
|
|
|
|
|
||||||||
|
|
|
|
|
|
|
|
|
|
j In |
|
|
|
|
|
|
|
|
|
|
|
|
|
and d |
are the vectors of volume fractions, porosity, and sizes of |
||||||||||||||||||
where |
, q |
particles of disperse component fractions, respectively, in the polymer composition; optj are the optimum volume fractions of filler in the composition; φjv is
the volume fraction of a v th fraction of a j th kind of filler in the composition; mj is the number of fractions of the j th disperse component; minj and maxj are
the lower and upper limits for the volume fractions of solid components in the composition; xoptj is the mass concentration of solid disperse components in
the polymer composition, optimum for the corresponding block of characteristics, for example, mechanical ones; γj is the density of solid disperse components; In is a set of indices belonging to the kinds of filler (for example, alumina and silica) entering into the formulation of the composite.
70