
- •Contents
- •Symbols and Abbreviations
- •Symbols
- •Greek Symbols
- •Subscripts
- •Abbreviations
- •Preface
- •Road Map of the Book
- •The Arrangement
- •Suggested Route for the Coursework
- •First Semester
- •Second Semester
- •Suggestions for the Class
- •Use of Semi-empirical Relations
- •1 Introduction
- •1.1 Overview
- •1.1.1 What Is to Be Learned?
- •1.1.2 Coursework Content
- •1.2 Brief Historical Background
- •1.3 Current Aircraft Design Status
- •1.3.1 Forces and Drivers
- •1.3.2 Current Civil Aircraft Design Trends
- •1.3.3 Current Military Aircraft Design Trends
- •1.4 Future Trends
- •1.4.1 Civil Aircraft Design: Future Trends
- •1.4.2 Military Aircraft Design: Future Trends
- •1.5 Learning Process
- •1.6 Units and Dimensions
- •1.7 Cost Implications
- •2 Methodology to Aircraft Design, Market Survey, and Airworthiness
- •2.1 Overview
- •2.1.1 What Is to Be Learned?
- •2.1.2 Coursework Content
- •2.2 Introduction
- •2.3 Typical Design Process
- •2.3.1 Four Phases of Aircraft Design
- •2.3.2 Typical Resources Deployment
- •2.3.3 Typical Cost Frame
- •2.3.4 Typical Time Frame
- •2.4 Typical Task Breakdown in Each Phase
- •Phase 1: Conceptual Study Phase (Feasibility Study)
- •Phase 3: Detailed Design Phase (Full-Scale Product Development)
- •2.4.1 Functional Tasks during the Conceptual Study (Phase 1: Civil Aircraft)
- •2.4.2 Project Activities for Small Aircraft Design
- •Phase 1: Conceptual Design (6 Months)
- •Phase 3: Detailed Design (Product Development) (12 Months)
- •2.5 Aircraft Familiarization
- •Fuselage Group
- •Wing Group
- •Empennage Group
- •Nacelle Group
- •Undercarriage Group
- •2.6 Market Survey
- •2.7 Civil Aircraft Market
- •2.8 Military Market
- •2.9 Comparison between Civil and Military Aircraft Design Requirements
- •2.10 Airworthiness Requirements
- •2.11 Coursework Procedures
- •3 Aerodynamic Considerations
- •3.1 Overview
- •3.1.1 What Is to Be Learned?
- •3.1.2 Coursework Content
- •3.2 Introduction
- •3.3 Atmosphere
- •3.4 Fundamental Equations
- •3.5.1 Flow Past Aerofoil
- •3.6 Aircraft Motion and Forces
- •3.6.1 Motion
- •3.6.2 Forces
- •3.7 Aerofoil
- •3.7.1 Groupings of Aerofoils and Their Properties
- •NACA Four-Digit Aerofoil
- •NACA Five-Digit Aerofoil
- •NACA Six-Digit Aerofoil
- •Other Types of Aerofoils
- •3.9 Generation of Lift
- •3.10 Types of Stall
- •3.10.1 Gradual Stall
- •3.10.2 Abrupt Stall
- •3.11 Comparison of Three NACA Aerofoils
- •3.12 High-Lift Devices
- •3.13 Transonic Effects – Area Rule
- •3.14 Wing Aerodynamics
- •3.14.1 Induced Drag and Total Aircraft Drag
- •3.15 Aspect Ratio Correction of 2D Aerofoil Characteristics for 3D Finite Wing
- •3.16.1 Planform Area, SW
- •3.16.2 Wing Aspect Ratio
- •3.16.4 Wing Root (Croot) and Tip (Ctip) Chord
- •3.16.6 Wing Twist
- •3.17 Mean Aerodynamic Chord
- •3.18 Compressibility Effect: Wing Sweep
- •3.19 Wing Stall Pattern and Wing Twist
- •3.20.1 The Square-Cube Law
- •3.20.2 Aircraft Wetted Area (AW) versus Wing Planform Area (Sw)
- •3.20.3 Additional Vortex Lift
- •3.20.4 Additional Surfaces on Wing
- •3.21 Finalizing Wing Design Parameters
- •3.22 Empennage
- •3.22.1 H-Tail
- •3.22.2 V-Tail
- •3.23 Fuselage
- •3.23.2 Fuselage Length, Lfus
- •3.23.3 Fineness Ratio, FR
- •3.23.4 Fuselage Upsweep Angle
- •3.23.5 Fuselage Closure Angle
- •3.23.6 Front Fuselage Closure Length, Lf
- •3.23.7 Aft Fuselage Closure Length, La
- •3.23.8 Midfuselage Constant Cross-Section Length, Lm
- •3.23.9 Fuselage Height, H
- •3.23.10 Fuselage Width, W
- •3.23.11 Average Diameter, Dave
- •3.23.12 Cabin Height, Hcab
- •3.23.13 Cabin Width, Wcab
- •3.24 Undercarriage
- •3.25 Nacelle and Intake
- •3.26 Speed Brakes and Dive Brakes
- •4.1 Overview
- •4.1.1 What Is to Be Learned?
- •4.1.2 Coursework Content
- •4.2 Introduction
- •4.3 Aircraft Evolution
- •4.4 Civil Aircraft Mission (Payload-Range)
- •4.5 Civil Subsonic Jet Aircraft Statistics (Sizing Parameters and Regression Analysis)
- •4.5.1 Maximum Takeoff Mass versus Number of Passengers
- •4.5.2 Maximum Takeoff Mass versus Operational Empty Mass
- •4.5.3 Maximum Takeoff Mass versus Fuel Load
- •4.5.4 Maximum Takeoff Mass versus Wing Area
- •4.5.5 Maximum Takeoff Mass versus Engine Power
- •4.5.6 Empennage Area versus Wing Area
- •4.5.7 Wing Loading versus Aircraft Span
- •4.6 Civil Aircraft Component Geometries
- •4.7 Fuselage Group
- •4.7.1 Fuselage Width
- •4.7.2 Fuselage Length
- •4.7.3 Front (Nose Cone) and Aft-End Closure
- •4.7.4 Flight Crew (Flight Deck) Compartment Layout
- •4.7.5 Cabin Crew and Passenger Facilities
- •4.7.6 Seat Arrangement, Pitch, and Posture (95th Percentile) Facilities
- •4.7.7 Passenger Facilities
- •4.7.8 Cargo Container Sizes
- •4.7.9 Doors – Emergency Exits
- •4.8 Wing Group
- •4.9 Empennage Group (Civil Aircraft)
- •4.10 Nacelle Group
- •4.11 Summary of Civil Aircraft Design Choices
- •4.13 Military Aircraft Mission
- •4.14.1 Military Aircraft Maximum Take-off Mass (MTOM) versus Payload
- •4.14.2 Military MTOM versus OEM
- •4.14.3 Military MTOM versus Fuel Load Mf
- •4.14.4 MTOM versus Wing Area (Military)
- •4.14.5 MTOM versus Engine Thrust (Military)
- •4.14.6 Empennage Area versus Wing Area (Military)
- •4.14.7 Aircraft Wetted Area versus Wing Area (Military)
- •4.15 Military Aircraft Component Geometries
- •4.16 Fuselage Group (Military)
- •4.17 Wing Group (Military)
- •4.17.1 Generic Wing Planform Shapes
- •4.18 Empennage Group (Military)
- •4.19 Intake/Nacelle Group (Military)
- •4.20 Undercarriage Group
- •4.21 Miscellaneous Comments
- •4.22 Summary of Military Aircraft Design Choices
- •5 Aircraft Load
- •5.1 Overview
- •5.1.1 What Is to Be Learned?
- •5.1.2 Coursework Content
- •5.2 Introduction
- •5.2.1 Buffet
- •5.2.2 Flutter
- •5.3 Flight Maneuvers
- •5.3.1 Pitch Plane (X-Z) Maneuver (Elevator/Canard-Induced)
- •5.3.2 Roll Plane (Y-Z) Maneuver (Aileron-Induced)
- •5.3.3 Yaw Plane (Z-X) Maneuver (Rudder-Induced)
- •5.4 Aircraft Loads
- •5.4.1 On the Ground
- •5.4.2 In Flight
- •5.5.1 Load Factor, n
- •5.6 Limits – Load and Speeds
- •5.6.1 Maximum Limit of Load Factor
- •5.6.2 Speed Limits
- •5.7 V-n Diagram
- •5.7.1 Low-Speed Limit
- •5.7.2 High-Speed Limit
- •5.7.3 Extreme Points of a V-n Diagram
- •Positive Loads
- •Negative Loads
- •5.8 Gust Envelope
- •6.1 Overview
- •6.1.1 What Is to Be Learned?
- •6.1.2 Coursework Content
- •6.2 Introduction
- •Closure of the Fuselage
- •6.4 Civil Aircraft Fuselage: Typical Shaping and Layout
- •6.4.1 Narrow-Body, Single-Aisle Aircraft
- •6.4.2 Wide-Body, Double-Aisle Aircraft
- •6.4.3 Worked-Out Example: Civil Aircraft Fuselage Layout
- •6.5.1 Aerofoil Selection
- •6.5.2 Wing Design
- •Planform Shape
- •Wing Reference Area
- •Wing Sweep
- •Wing Twist
- •Wing Dihedral/Anhedral
- •6.5.3 Wing-Mounted Control-Surface Layout
- •6.5.4 Positioning of the Wing Relative to the Fuselage
- •6.6.1 Horizontal Tail
- •6.6.2 Vertical Tail
- •6.8 Undercarriage Positioning
- •6.10 Miscellaneous Considerations in Civil Aircraft
- •6.12.1 Use of Statistics in the Class of Military Trainer Aircraft
- •6.12.3 Miscellaneous Considerations – Military Design
- •6.13 Variant CAS Design
- •6.13.1 Summary of the Worked-Out Military Aircraft Preliminary Details
- •7 Undercarriage
- •7.1 Overview
- •7.1.1 What Is to Be Learned?
- •7.1.2 Coursework Content
- •7.2 Introduction
- •7.3 Types of Undercarriage
- •7.5 Undercarriage Retraction and Stowage
- •7.5.1 Stowage Space Clearances
- •7.6 Undercarriage Design Drivers and Considerations
- •7.7 Turning of an Aircraft
- •7.8 Wheels
- •7.9 Loads on Wheels and Shock Absorbers
- •7.9.1 Load on Wheels
- •7.9.2 Energy Absorbed
- •7.11 Tires
- •7.13 Undercarriage Layout Methodology
- •7.14 Worked-Out Examples
- •7.14.1 Civil Aircraft: Bizjet
- •Baseline Aircraft with 10 Passengers at a 33-Inch Pitch
- •Shrunk Aircraft (Smallest in the Family Variant) with 6 Passengers at a 33-Inch Pitch
- •7.14.2 Military Aircraft: AJT
- •7.15 Miscellaneous Considerations
- •7.16 Undercarriage and Tire Data
- •8 Aircraft Weight and Center of Gravity Estimation
- •8.1 Overview
- •8.1.1 What Is to Be Learned?
- •8.1.2 Coursework Content
- •8.2 Introduction
- •8.3 The Weight Drivers
- •8.4 Aircraft Mass (Weight) Breakdown
- •8.5 Desirable CG Position
- •8.6 Aircraft Component Groups
- •8.6.1 Civil Aircraft
- •8.6.2 Military Aircraft (Combat Category)
- •8.7 Aircraft Component Mass Estimation
- •8.8 Rapid Mass Estimation Method: Civil Aircraft
- •8.9 Graphical Method for Predicting Aircraft Component Weight: Civil Aircraft
- •8.10 Semi-empirical Equation Method (Statistical)
- •8.10.1 Fuselage Group – Civil Aircraft
- •8.10.2 Wing Group – Civil Aircraft
- •8.10.3 Empennage Group – Civil Aircraft
- •8.10.4 Nacelle Group – Civil Aircraft
- •Jet Type (Includes Pylon Mass)
- •Turboprop Type
- •Piston-Engine Nacelle
- •8.10.5 Undercarriage Group – Civil Aircraft
- •Tricycle Type (Retractable) – Fuselage-Mounted (Nose and Main Gear Estimated Together)
- •8.10.6 Miscellaneous Group – Civil Aircraft
- •8.10.7 Power Plant Group – Civil Aircraft
- •Turbofans
- •Turboprops
- •Piston Engines
- •8.10.8 Systems Group – Civil Aircraft
- •8.10.9 Furnishing Group – Civil Aircraft
- •8.10.10 Contingency and Miscellaneous – Civil Aircraft
- •8.10.11 Crew – Civil Aircraft
- •8.10.12 Payload – Civil Aircraft
- •8.10.13 Fuel – Civil Aircraft
- •8.11 Worked-Out Example – Civil Aircraft
- •8.11.1 Fuselage Group Mass
- •8.11.2 Wing Group Mass
- •8.11.3 Empennage Group Mass
- •8.11.4 Nacelle Group Mass
- •8.11.5 Undercarriage Group Mass
- •8.11.6 Miscellaneous Group Mass
- •8.11.7 Power Plant Group Mass
- •8.11.8 Systems Group Mass
- •8.11.9 Furnishing Group Mass
- •8.11.10 Contingency Group Mass
- •8.11.11 Crew Mass
- •8.11.12 Payload Mass
- •8.11.13 Fuel Mass
- •8.11.14 Weight Summary
- •Variant Aircraft in the Family
- •8.12 Center of Gravity Determination
- •8.12.1 Bizjet Aircraft CG Location Example
- •8.12.2 First Iteration to Fine Tune CG Position Relative to Aircraft and Components
- •8.13 Rapid Mass Estimation Method – Military Aircraft
- •8.14 Graphical Method to Predict Aircraft Component Weight – Military Aircraft
- •8.15 Semi-empirical Equation Methods (Statistical) – Military Aircraft
- •8.15.1 Military Aircraft Fuselage Group (SI System)
- •8.15.2 Military Aircraft Wing Mass (SI System)
- •8.15.3 Military Aircraft Empennage
- •8.15.4 Nacelle Mass Example – Military Aircraft
- •8.15.5 Power Plant Group Mass Example – Military Aircraft
- •8.15.6 Undercarriage Mass Example – Military Aircraft
- •8.15.7 System Mass – Military Aircraft
- •8.15.8 Aircraft Furnishing – Military Aircraft
- •8.15.11 Crew Mass
- •8.16.1 AJT Fuselage Example (Based on CAS Variant)
- •8.16.2 AJT Wing Example (Based on CAS Variant)
- •8.16.3 AJT Empennage Example (Based on CAS Variant)
- •8.16.4 AJT Nacelle Mass Example (Based on CAS Variant)
- •8.16.5 AJT Power Plant Group Mass Example (Based on AJT Variant)
- •8.16.6 AJT Undercarriage Mass Example (Based on CAS Variant)
- •8.16.7 AJT Systems Group Mass Example (Based on AJT Variant)
- •8.16.8 AJT Furnishing Group Mass Example (Based on AJT Variant)
- •8.16.9 AJT Contingency Group Mass Example
- •8.16.10 AJT Crew Mass Example
- •8.16.13 Weights Summary – Military Aircraft
- •8.17 CG Position Determination – Military Aircraft
- •8.17.1 Classroom Worked-Out Military AJT CG Location Example
- •8.17.2 First Iteration to Fine Tune CG Position and Components Masses
- •9 Aircraft Drag
- •9.1 Overview
- •9.1.1 What Is to Be Learned?
- •9.1.2 Coursework Content
- •9.2 Introduction
- •9.4 Aircraft Drag Breakdown (Subsonic)
- •9.5 Aircraft Drag Formulation
- •9.6 Aircraft Drag Estimation Methodology (Subsonic)
- •9.7 Minimum Parasite Drag Estimation Methodology
- •9.7.2 Computation of Wetted Areas
- •Lifting Surfaces
- •Fuselage
- •Nacelle
- •9.7.3 Stepwise Approach to Compute Minimum Parasite Drag
- •9.8 Semi-empirical Relations to Estimate Aircraft Component Parasite Drag
- •9.8.1 Fuselage
- •9.8.2 Wing, Empennage, Pylons, and Winglets
- •9.8.3 Nacelle Drag
- •Intake Drag
- •Base Drag
- •Boat-Tail Drag
- •9.8.4 Excrescence Drag
- •9.8.5 Miscellaneous Parasite Drags
- •Air-Conditioning Drag
- •Trim Drag
- •Aerials
- •9.9 Notes on Excrescence Drag Resulting from Surface Imperfections
- •9.10 Minimum Parasite Drag
- •9.12 Subsonic Wave Drag
- •9.13 Total Aircraft Drag
- •9.14 Low-Speed Aircraft Drag at Takeoff and Landing
- •9.14.1 High-Lift Device Drag
- •9.14.2 Dive Brakes and Spoilers Drag
- •9.14.3 Undercarriage Drag
- •9.14.4 One-Engine Inoperative Drag
- •9.15 Propeller-Driven Aircraft Drag
- •9.16 Military Aircraft Drag
- •9.17 Supersonic Drag
- •9.18 Coursework Example: Civil Bizjet Aircraft
- •9.18.1 Geometric and Performance Data
- •Fuselage (see Figure 9.13)
- •Wing (see Figure 9.13)
- •Empennage (see Figure 9.13)
- •Nacelle (see Figure 9.13)
- •9.18.2 Computation of Wetted Areas, Re, and Basic CF
- •Fuselage
- •Wing
- •Empennage (same procedure as for the wing)
- •Nacelle
- •Pylon
- •9.18.3 Computation of 3D and Other Effects to Estimate Component
- •Fuselage
- •Wing
- •Empennage
- •Nacelle
- •Pylon
- •9.18.4 Summary of Parasite Drag
- •9.18.5 CDp Estimation
- •9.18.6 Induced Drag
- •9.18.7 Total Aircraft Drag at LRC
- •9.19 Coursework Example: Subsonic Military Aircraft
- •9.19.1 Geometric and Performance Data of a Vigilante RA-C5 Aircraft
- •Fuselage
- •Wing
- •Empennage
- •9.19.2 Computation of Wetted Areas, Re, and Basic CF
- •Fuselage
- •Wing
- •Empennage (same procedure as for the wing)
- •9.19.3 Computation of 3D and Other Effects to Estimate Component CDpmin
- •Fuselage
- •Wing
- •Empennage
- •9.19.4 Summary of Parasite Drag
- •9.19.5 CDp Estimation
- •9.19.6 Induced Drag
- •9.19.7 Supersonic Drag Estimation
- •9.19.8 Total Aircraft Drag
- •9.20 Concluding Remarks
- •10 Aircraft Power Plant and Integration
- •10.1 Overview
- •10.1.1 What Is to Be Learned?
- •10.1.2 Coursework Content
- •10.2 Background
- •10.4 Introduction: Air-Breathing Aircraft Engine Types
- •10.4.1 Simple Straight-Through Turbojet
- •10.4.2 Turbofan: Bypass Engine
- •10.4.3 Afterburner Engine
- •10.4.4 Turboprop Engine
- •10.4.5 Piston Engine
- •10.6 Formulation and Theory: Isentropic Case
- •10.6.1 Simple Straight-Through Turbojet Engine: Formulation
- •10.6.2 Bypass Turbofan Engine: Formulation
- •10.6.3 Afterburner Engine: Formulation
- •10.6.4 Turboprop Engine: Formulation
- •Summary
- •10.7 Engine Integration with an Aircraft: Installation Effects
- •10.7.1 Subsonic Civil Aircraft Nacelle and Engine Installation
- •10.7.2 Turboprop Integration to Aircraft
- •10.7.3 Combat Aircraft Engine Installation
- •10.8 Intake and Nozzle Design
- •10.8.1 Civil Aircraft Intake Design: Inlet Sizing
- •10.8.2 Military Aircraft Intake Design
- •10.9 Exhaust Nozzle and Thrust Reverser
- •10.9.1 Civil Aircraft Thrust Reverser Application
- •10.9.2 Civil Aircraft Exhaust Nozzles
- •10.9.3 Coursework Example of Civil Aircraft Nacelle Design
- •Intake Geometry (see Section 10.8.1)
- •Lip Section (Crown Cut)
- •Lip Section (Keel Cut)
- •Nozzle Geometry
- •10.9.4 Military Aircraft Thrust Reverser Application and Exhaust Nozzles
- •10.10 Propeller
- •10.10.2 Propeller Theory
- •Momentum Theory: Actuator Disc
- •Blade-Element Theory
- •10.10.3 Propeller Performance: Practical Engineering Applications
- •Static Performance (see Figures 10.34 and 10.36)
- •In-Flight Performance (see Figures 10.35 and 10.37)
- •10.10.5 Propeller Performance at STD Day: Worked-Out Example
- •10.11 Engine-Performance Data
- •Takeoff Rating
- •Maximum Continuous Rating
- •Maximum Climb Rating
- •Maximum Cruise Rating
- •Idle Rating
- •10.11.1 Piston Engine
- •10.11.2 Turboprop Engine (Up to 100 Passengers Class)
- •Takeoff Rating
- •Maximum Climb Rating
- •Maximum Cruise Rating
- •10.11.3 Turbofan Engine: Civil Aircraft
- •Turbofans with a BPR Around 4 (Smaller Engines; e.g., Bizjets)
- •Turbofans with a BPR around 5 or 7 (Larger Engines; e.g., RJs and Larger)
- •10.11.4 Turbofan Engine – Military Aircraft
- •11 Aircraft Sizing, Engine Matching, and Variant Derivative
- •11.1 Overview
- •11.1.1 What Is to Be Learned?
- •11.1.2 Coursework Content
- •11.2 Introduction
- •11.3 Theory
- •11.3.1 Sizing for Takeoff Field Length
- •Civil Aircraft Design: Takeoff
- •Military Aircraft Design: Takeoff
- •11.3.2 Sizing for the Initial Rate of Climb
- •11.3.3 Sizing to Meet Initial Cruise
- •11.3.4 Sizing for Landing Distance
- •11.4 Coursework Exercises: Civil Aircraft Design (Bizjet)
- •11.4.1 Takeoff
- •11.4.2 Initial Climb
- •11.4.3 Cruise
- •11.4.4 Landing
- •11.5 Coursework Exercises: Military Aircraft Design (AJT)
- •11.5.1 Takeoff – Military Aircraft
- •11.5.2 Initial Climb – Military Aircraft
- •11.5.3 Cruise – Military Aircraft
- •11.5.4 Landing – Military Aircraft
- •11.6 Sizing Analysis: Civil Aircraft (Bizjet)
- •11.6.1 Variants in the Family of Aircraft Design
- •11.6.2 Example: Civil Aircraft
- •11.7 Sizing Analysis: Military Aircraft
- •11.7.1 Single-Seat Variant in the Family of Aircraft Design
- •11.8 Sensitivity Study
- •11.9 Future Growth Potential
- •12.1 Overview
- •12.1.1 What Is to Be Learned?
- •12.1.2 Coursework Content
- •12.2 Introduction
- •12.3 Static and Dynamic Stability
- •12.3.1 Longitudinal Stability: Pitch Plane (Pitch Moment, M)
- •12.3.2 Directional Stability: Yaw Plane (Yaw Moment, N)
- •12.3.3 Lateral Stability: Roll Plane (Roll Moment, L)
- •12.3.4 Summary of Forces, Moments, and Their Sign Conventions
- •12.4 Theory
- •12.4.1 Pitch Plane
- •12.4.2 Yaw Plane
- •12.4.3 Roll Plane
- •12.6 Inherent Aircraft Motions as Characteristics of Design
- •12.6.1 Short-Period Oscillation and Phugoid Motion
- •12.6.2 Directional and Lateral Modes of Motion
- •12.7 Spinning
- •12.8 Design Considerations for Stability: Civil Aircraft
- •12.9 Military Aircraft: Nonlinear Effects
- •12.10 Active Control Technology: Fly-by-Wire
- •13 Aircraft Performance
- •13.1 Overview
- •13.1.1 What Is to Be Learned?
- •13.1.2 Coursework Content
- •13.2 Introduction
- •13.2.1 Aircraft Speed
- •13.3 Establish Engine Performance Data
- •13.3.1 Turbofan Engine (BPR < 4)
- •Takeoff Rating (Bizjet): Standard Day
- •Maximum Climb Rating (Bizjet): Standard Day
- •Maximum Cruise Rating (Bizjet): Standard Day
- •13.3.2 Turbofan Engine (BPR > 4)
- •13.3.3 Military Turbofan (Advanced Jet Trainer/CAS Role – Very Low BPR) – STD Day
- •13.3.4 Turboprop Engine Performance
- •Takeoff Rating (Turboprop): Standard Day
- •Maximum Climb Rating (Turboprop): Standard Day
- •Maximum Cruise Rating (Turboprop): Standard Day
- •13.4 Derivation of Pertinent Aircraft Performance Equations
- •13.4.1 Takeoff
- •Balanced Field Length: Civil Aircraft
- •Takeoff Equations
- •13.4.2 Landing Performance
- •13.4.3 Climb and Descent Performance
- •Summary
- •Descent
- •13.4.4 Initial Maximum Cruise Speed
- •13.4.5 Payload Range Capability
- •13.5 Aircraft Performance Substantiation: Worked-Out Examples (Bizjet)
- •13.5.1 Takeoff Field Length (Bizjet)
- •Segment A: All Engines Operating up to the Decision Speed V1
- •Segment B: One-Engine Inoperative Acceleration from V1 to Liftoff Speed, VLO
- •Segment C: Flaring Distance with One Engine Inoperative from VLO to V2
- •Segment E: Braking Distance from VB to Zero Velocity (Flap Settings Are of Minor Consequence)
- •Discussion of the Takeoff Analysis
- •13.5.2 Landing Field Length (Bizjet)
- •13.5.3 Climb Performance Requirements (Bizjet)
- •13.5.4 Integrated Climb Performance (Bizjet)
- •13.5.5 Initial High-Speed Cruise (Bizjet)
- •13.5.7 Descent Performance (Bizjet)
- •13.5.8 Payload Range Capability
- •13.6 Aircraft Performance Substantiation: Military Aircraft (AJT)
- •13.6.2 Takeoff Field Length (AJT)
- •Distance Covered from Zero to the Decision Speed V1
- •Distance Covered from Zero to Liftoff Speed VLO
- •Distance Covered from VLO to V2
- •Total Takeoff Distance
- •Stopping Distance and the CFL
- •Distance Covered from V1 to Braking Speed VB
- •Verifying the Climb Gradient at an 8-Deg Flap
- •13.6.3 Landing Field Length (AJT)
- •13.6.4 Climb Performance Requirements (AJT)
- •13.6.5 Maximum Speed Requirements (AJT)
- •13.6.6 Fuel Requirements (AJT)
- •13.7 Summary
- •13.7.1 The Bizjet
- •14 Computational Fluid Dynamics
- •14.1 Overview
- •14.1.1 What Is to Be Learned?
- •14.1.2 Coursework Content
- •14.2 Introduction
- •14.3 Current Status
- •14.4 Approach to CFD Analyses
- •14.4.1 In the Preprocessor (Menu-Driven)
- •14.4.2 In the Flow Solver (Menu-Driven)
- •14.4.3 In the Postprocessor (Menu-Driven)
- •14.5 Case Studies
- •14.6 Hierarchy of CFD Simulation Methods
- •14.6.1 DNS Simulation Technique
- •14.6.2 Large Eddy Simulation (LES) Technique
- •14.6.3 Detached Eddy Simulation (DES) Technique
- •14.6.4 RANS Equation Technique
- •14.6.5 Euler Method Technique
- •14.6.6 Full-Potential Flow Equations
- •14.6.7 Panel Method
- •14.7 Summary
- •15 Miscellaneous Design Considerations
- •15.1 Overview
- •15.1.1 What Is to Be Learned?
- •15.1.2 Coursework Content
- •15.2 Introduction
- •15.2.1 Environmental Issues
- •15.2.2 Materials and Structures
- •15.2.3 Safety Issues
- •15.2.4 Human Interface
- •15.2.5 Systems Architecture
- •15.2.6 Military Aircraft Survivability Issues
- •15.2.7 Emerging Scenarios
- •15.3 Noise Emissions
- •Approach
- •Sideline
- •15.3.1 Summary
- •15.4 Engine Exhaust Emissions
- •15.5 Aircraft Materials
- •15.5.1 Material Properties
- •15.5.2 Material Selection
- •15.5.3 Coursework Overview
- •Civil Aircraft Design
- •Military Aircraft Design
- •15.6 Aircraft Structural Considerations
- •15.7 Doors: Emergency Egress
- •Coursework Exercise
- •15.8 Aircraft Flight Deck (Cockpit) Layout
- •15.8.1 Multifunctional Display and Electronic Flight Information System
- •15.8.2 Combat Aircraft Flight Deck
- •15.8.3 Civil Aircraft Flight Deck
- •15.8.4 Head-Up Display
- •15.8.5 Helmet-Mounted Display
- •15.8.6 Hands-On Throttle and Stick
- •15.8.7 Voice-Operated Control
- •15.9 Aircraft Systems
- •15.9.1 Aircraft Control Subsystem
- •15.9.2 Engine and Fuel Control Subsystems
- •Piston Engine Fuel Control System (The total system weight is approximately 1 to 1.5% of the MTOW)
- •Turbofan Engine Fuel Control System (The total system weight is approximately 1.5 to 2% of the MTOW)
- •Fuel Storage and Flow Management
- •15.9.3 Emergency Power Supply
- •15.9.4 Avionics Subsystems
- •Military Aircraft Application
- •Civil Aircraft Application
- •15.9.5 Electrical Subsystem
- •15.9.6 Hydraulic Subsystem
- •15.9.7 Pneumatic System
- •ECS: Cabin Pressurization and Air-Conditioning
- •Oxygen Supply
- •Anti-icing, De-icing, Defogging, and Rain-Removal Systems
- •Defogging and Rain-Removal Systems
- •15.9.8 Utility Subsystem
- •15.9.9 End-of-Life Disposal
- •15.10 Military Aircraft Survivability
- •15.10.1 Military Emergency Escape
- •15.10.2 Military Aircraft Stealth Consideration
- •15.11 Emerging Scenarios
- •Counterterrorism Design Implementation
- •Health Issues
- •Damage from Runway Debris
- •16 Aircraft Cost Considerations
- •16.1 Overview
- •16.1.1 What Is to Be Learned?
- •16.1.2 Coursework Content
- •16.2 Introduction
- •16.3 Aircraft Cost and Operational Cost
- •Operating Cost
- •16.4 Aircraft Costing Methodology: Rapid-Cost Model
- •16.4.1 Nacelle Cost Drivers
- •Group 1
- •Group 2
- •16.4.2 Nose Cowl Parts and Subassemblies
- •16.4.3 Methodology (Nose Cowl Only)
- •Cost of Parts Fabrication
- •Subassemblies
- •Cost of Amortization of the NRCs
- •16.4.4 Cost Formulas and Results
- •16.5 Aircraft Direct Operating Cost
- •16.5.1 Formulation to Estimate DOC
- •Aircraft Price
- •Fixed-Cost Elements
- •Trip-Cost Elements
- •16.5.2 Worked-Out Example of DOC: Bizjet
- •Aircraft Price
- •Fixed-Cost Elements
- •Trip-Cost Elements
- •OC of the Variants in the Family
- •17 Aircraft Manufacturing Considerations
- •17.1 Overview
- •17.1.1 What Is to Be Learned?
- •17.1.2 Coursework Content
- •17.2 Introduction
- •17.3 Design for Manufacture and Assembly
- •17.4 Manufacturing Practices
- •17.5 Six Sigma Concept
- •17.6 Tolerance Relaxation at the Wetted Surface
- •17.6.1 Sources of Aircraft Surface Degeneration
- •17.6.2 Cost-versus-Tolerance Relationship
- •17.7 Reliability and Maintainability
- •17.8 Design Considerations
- •17.8.1 Category I: Technology-Driven Design Considerations
- •17.8.2 Category II: Manufacture-Driven Design Considerations
- •17.8.3 Category III: Management-Driven Design Considerations
- •17.8.4 Category IV: Operator-Driven Design Considerations
- •17.9 “Design for Customer”
- •17.9.1 Index for “Design for Customer”
- •17.9.2 Worked-Out Example
- •Standard Parameters of the Baseline Aircraft
- •Parameters of the Extended Variant Aircraft
- •Parameters of the Shortened Variant Aircraft
- •17.10 Digital Manufacturing Process Management
- •Process Detailing and Validation
- •Resource Modeling and Simulation
- •Process Planning and Simulation
- •17.10.1 Product, Process, and Resource Hub
- •17.10.3 Shop-Floor Interface
- •17.10.4 Design for Maintainability and 3D-Based Technical Publication Generation
- •Midrange Aircraft (Airbus 320 class)
- •References
- •ROAD MAP OF THE BOOK
- •CHAPTER 1. INTRODUCTION
- •CHAPTER 3. AERODYNAMIC CONSIDERATIONS
- •CHAPTER 5. AIRCRAFT LOAD
- •CHAPTER 6. CONFIGURING AIRCRAFT
- •CHAPTER 7. UNDERCARRIAGE
- •CHAPTER 8. AIRCRAFT WEIGHT AND CENTER OF GRAVITY ESTIMATION
- •CHAPTER 9. AIRCRAFT DRAG
- •CHAPTER 10. AIRCRAFT POWER PLANT AND INTEGRATION
- •CHAPTER 11. AIRCRAFT SIZING, ENGINE MATCHING, AND VARIANT DERIVATIVE
- •CHAPTER 12. STABILITY CONSIDERATIONS AFFECTING AIRCRAFT CONFIGURATION
- •CHAPTER 13. AIRCRAFT PERFORMANCE
- •CHAPTER 14. COMPUTATIONAL FLUID DYNAMICS
- •CHAPTER 15. MISCELLANEOUS DESIGN CONSIDERATIONS
- •CHAPTER 16. AIRCRAFT COST CONSIDERATIONS
- •CHAPTER 17. AIRCRAFT MANUFACTURING CONSIDERATIONS
- •Index
114 |
Aircraft Classification, Statistics, and Choices for Configuration |
passenger loading so that the longest in an aircraft family does not exceed the fineness ratio on the order of 13. The appropriate front and aft-end closure choices are then made. When the fuselage shell is established, the next task is to configure the interior for passenger and crew requirements. The flightcrew space in the forward closure (i.e., cockpit) and the pilot vision polar are then established. Inside the cabin, the crew and passenger requirements are approached simultaneously as integral requirements (e.g., seating, toilets, and galleys).
2.Wing Group. This is the most important component of the aircraft. The planform shape must be established and then sized for operational-field and flightperformance requirements. Options for high-lift devices are described in Section 3.12. Other smaller components (e.g., winglets) also are considered (see Section 3.21) but not all aircraft incorporate winglets.
3.Empennage Group. Choice, size, and placement result from the aircraft’s CG position and wing size. This book adheres to the conventional H-tail and V-tail configuration.
4.Nacelle Group. This topic is addressed in Chapter 10; only an outline for the shaping choice is provided herein.
These four groups of aircraft components provide the preliminary shaping of candidate aircraft configurations. After the wing-sizing and engine-matching exercises, the choice must be narrowed to one final configuration that offers the best compromise for the family variants to cover a wide market. The undercarriage is addressed separately in Chapter 7.
Iterations are required to position the empennage and undercarriage with respect to the wing because the CG position initially is not known. Weights are estimated from a provisional positioning and then the positions are fine tuned through iterations. (In a classroom exercise, one iteration is sufficient.)
4.7 Fuselage Group
Fuselage geometry is determined from the designed passenger capacity (see Chapter 6). There are two parameters to size (i.e., fuselage width [W] and fuselage length [Lf]), which determine the constant-section fuselage-barrel length. In turn, this depends on the seat pitch and width for the desired passenger comfort level. Table 4.2 lists the statistics for existing designs – a new design would be similar. The width and length of the fuselage must be determined simultaneously, bearing in mind that the maximum growth potential in the family of variants cannot be too long or too short and keeping the fineness ratio from 7 to 14 (a good value is around 10). Boeing 757–300 records the highest fineness ratio of 14.7. A seating arrangement with two aisles results in more than six abreast (average diameter, Dave = [H + W]/2; see Figure 4.14).
4.7.1 Fuselage Width
The first parameter to determine for the fuselage average diameter is the number of abreast seating for passenger capacity. There is an overlap on choice for

4.7 Fuselage Group |
|
|
|
|
|
|
115 |
||
Table 4.2. Number of passengers versus number of abreast seating and fineness ratio |
|||||||||
|
|
|
|
|
|
|
|
||
|
|
|
|
|
|
|
|
||
Baseline |
Passenger |
Abreast |
Fuselage |
Length |
Fineness |
|
|
||
aircraft |
capacity |
seating |
Diaave -m |
m |
ratio |
Cross-section |
|||
|
|
|
|
|
|
|
|
|
|
Learjet45 |
6 (4 to 8) |
2 |
|
1.75 |
17.20 |
≈10.00 |
circular |
||
Dornier 228 |
18 |
2 |
|
|
|
≈ |
|
rectangular |
|
Dornier 328 |
24 |
3 |
|
2.20 |
20.92 |
≈ |
|
circular |
|
ERJ135 |
37 |
3 |
|
2.28 |
24.39 |
≈10.70 |
circular |
||
ERJ145 |
50 |
3 |
|
2.28 |
27.93 |
≈12.25 |
stretched version |
||
Canadair CL600 |
19 |
4 |
|
2.69 |
18.77 |
≈7.00 |
short fuselage |
||
Canadair RJ200 |
50 |
4 |
|
2.69 |
24.38 |
≈9.06 |
circular |
||
Canadair RJ900 |
86 |
4 |
|
2.69 |
36.16 |
≈13.44 |
stretched version |
||
Boeing 717–200 |
117 |
5 |
|
3.34 |
34.34 |
≈10.28 |
noncircular |
||
BAe145 (RJ100) |
100 |
5 |
|
3.56 |
30.00 |
≈8.43 |
|
|
|
Airbus 318 |
107 |
6 |
|
3.96 |
30.50 |
≈7.70 |
circular |
||
Airbus 321 |
185 |
6 |
|
3.96 |
44.00 |
≈11.10 |
circular |
||
Boeing 737–100 |
200 |
6 |
|
3.66 |
28.00 |
≈7.65 |
noncircular |
||
Boeing 737–900 |
200 |
6 |
|
3.66 |
42.11 |
≈11.50 |
family variant |
||
Boeing 757–300 |
230 |
6 |
|
3.66 |
54.00 |
≈14.70 |
highest ratio |
||
Boeing 767–300 |
260 |
7 |
|
5.03 |
53.67 |
≈10.70 |
circular |
||
Airbus 330–300 |
250 |
8 |
|
5.64 |
63.00 |
≈11.20 |
circular |
||
Airbus 340–600 |
380 |
8 |
|
5.64 |
75.30 |
≈13.35 |
circular |
||
Boeing 777–300 |
400 |
9 |
≈ |
6.20 |
73.86 |
≈11.90 |
circular |
||
Boeing 747–400 |
500 |
10 |
6.50 |
68.63 |
≈ |
10.55 |
partial double deck |
||
Airbus 380 |
600 |
10 |
≈ |
6.70 |
72.75 |
≈ |
10.80 |
full double deck |
|
|
|
More than 450-passenger capacity, the fuselage cross-section becomes a double-deck arrangement due to current restrictions of fuselage length to 80 m (262.5 ft). In the future, this restriction could be relaxed.
the midrange capacity in the family of design; for example, an A330 with 240 to 280 passengers has seven-abreast seating whereas the same passenger capacity in a B767 has eight-abreast seating. When seating number is increased to more than six abreast, the number of aisles is increased to two to alleviate congestion in passenger movement. Because of the current fuselage-length limitation of 80 m, largercapacity aircraft have a double-deck arrangement (e.g., the B747 and the A380). It would be interesting to try a two-aisle arrangement with six-abreast seating that would eliminate a middle seat. A three-aisle arrangement with ten-abreast seating would eliminate the cluster of four seats together. A BWB would have more than two aisles; there is no reason to not consider a triple-deck arrangement.
Although a circular cross-section is the most desirable relative to stress (minimize weight) and manufacture (minimize cost), the market requirements for the below-cabin floorspace arrangement could result in a cross-section elongated to an oval or elliptical shape. The Boeing 747 with a more narrow upper-deck width is a unique oval shape in the partial length that it extends. This partial length of the upper deck helps cross-sectional area distribution (see Section 3.23) and area ruling.
Figures 4.12 and 4.13 show various options for aircraft fuselage cross-sections to accommodate different seating arrangements. All fuselage cross-sections are symmetrical to the vertical plane. In general, aircraft with four-abreast seating and more have space below the cabin floor for baggage and cargo.
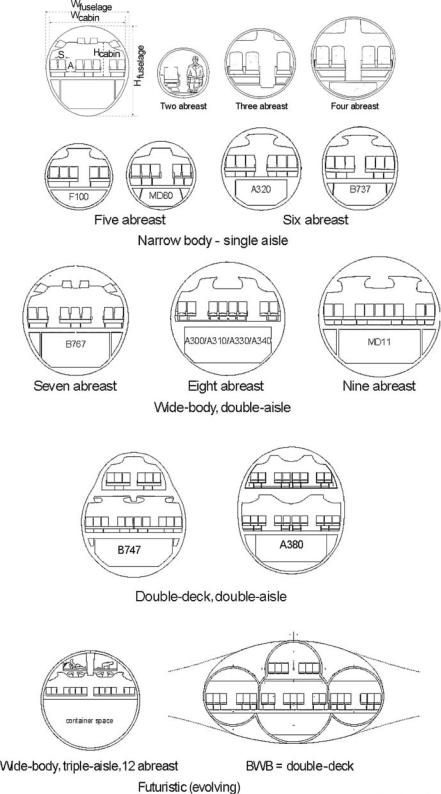
116 |
Aircraft Classification, Statistics, and Choices for Configuration |
Figure 4.12. Typical commercial transport aircraft fuselage cross-section (not to scale)

4.7 Fuselage Group |
117 |
Figure 4.13. Typical choices for the wing–fuselage position
Unpressurized propeller-driven aircraft operating at lower altitudes can have rectangular cross-sections to reduce manufacturing costs, as well as offer more space (e.g., Shorts 360 aircraft). A pressurized fuselage cross-section would invariably be circular or nearly circular to minimize weight from the point of hoop-stress considerations. A two-abreast circular cross-section would have cramped legroom; a better option is a slightly widened lower lobe (e.g., Learjet 45) to accommodate legroom. In general, with a three-passenger capacity and more, the midsection fuselage has a constant cross-section with front and aft ends tailored to suit the requirements. The wing box arrangement for smaller aircraft should pass over (e.g., high-wing DO328) (Figure 4.13) or under (e.g., Learjet 45) the fuselage.
4.7.2 Fuselage Length
The overall fuselage length, L (see Figure 3.49) consists of the (1) nose cone, (2) constant cross-section midsection barrel, and (3) aft-end closure. The constant crosssection mid-fuselage length is established from the passenger seating arrangement and combined with the class arrangement (i.e., first class, business class, and economy/tourist class). Section 4.7.6 provides seat dimensions for the two main classes (i.e., business and economy).
Aircraft length may not be equal to fuselage length if any other part of the aircraft extends beyond the fuselage extremities (e.g., the tail sweep may go beyond the tail cone of the fuselage; see Figure 6.8). Figure 4.14 shows the fuselage geometry relationship to the number of passengers. The fuselage width increases in increments with the number of passenger-abreast seating, one seat width at a time. Because of passenger comfort, a designer selects options from the sensitivity study (i.e., drag and cost variations); the continuous line in Figure 4.14 represents a typical average value. The actual width is determined in Chapter 6.
4.7.3 Front (Nose Cone) and Aft-End Closure
The tear-drop-shaped streamlined closure of the fuselage at both ends of the constant midsection keeps the nose cone blunter than the gradually tapered aft cone, as shown in Figure 4.15.

118 |
Aircraft Classification, Statistics, and Choices for Configuration |
Figure 4.14. Passenger number versus fuselage length (courtesy of MacMasters)
Figure 4.15 illustrates the front fuselage closure (i.e., nose cone) length, Lf, enclosing the flight deck (i.e., pilot cockpit), followed by the constant-section payload (passengers, in this case) shell. Being in a favorable pressure gradient of the flow, it is blunter than the aft closure. The aft-fuselage closure (tail cone) length, La, encloses the rear pressure bulkhead with a gradual closure in an adverse pressure gradient and has some degree of upsweep. In the center, the rotated cross-sectional view of the fuselage is shown.
average diameter, Dave = (H + W)/2 |
|
front-fuselage closure ratio, Fcf = Lf/Dave (also known as the |
(4.2) |
nose fineness ratio) |
|
aft-fuselage closure ratio, Fca = La/Dave |
|
Figure 4.16 shows several examples of current types of commercial transport aircraft designs. Statistical values for the frontand aft-fuselage closure are summarized in Table 4.3.
The front-end closure of bigger aircraft appears to be blunter than on smaller aircraft because the nose cone is sufficiently spacious to accommodate pilot positioning and instrumentation. A kink appears in the windscreen mould lines of the
Figure 4.15. Front (nose cone) and aft-end closure
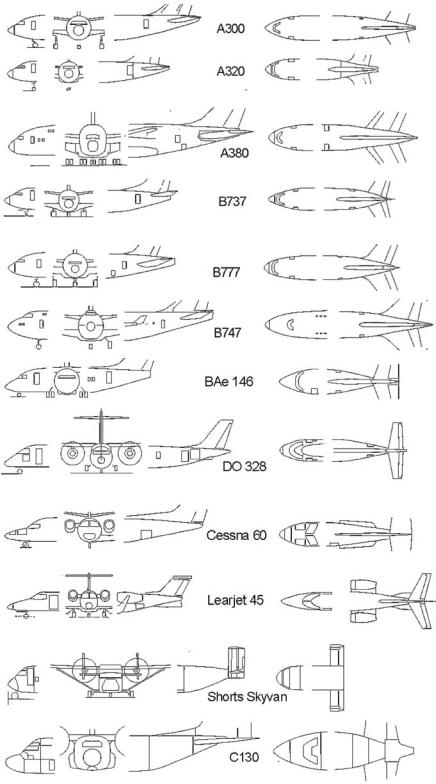
4.7 Fuselage Group |
119 |
Figure 4.16. Examples of several front and aft-end closure options (scale differs for each aircraft)

120 |
Aircraft Classification, Statistics, and Choices for Configuration |
|||||||||
Table 4.3. Fuselage closure parameters |
|
|
|
|
|
|
|
|
||
|
|
|
|
|
|
|
|
|
|
|
|
|
|
|
|
|
|
|
|
|
|
Aircraft |
L (m) |
D (m) |
H – (m) |
W – (m) |
H/W |
Lf/D |
La/D |
UA |
CA |
|
|
|
|
|
|
|
|
|
|
|
|
A300–600 (TA, TF, LW) |
53.62 |
5.64 |
5.64 |
5.64 |
1 |
2.60 |
3.103 |
5 |
9 |
|
A310–300 (TA, TF, LW) |
46.66 |
5.64 |
5.64 |
5.64 |
1 |
1.60 |
3.40 |
5 |
11 |
|
A320–200 (TA, TF, LW) |
37.57 |
3.96 |
3.96 |
3.96 |
1 |
1.50 |
3.40 |
4 |
8 |
|
A330–300 (TA, TF, LW) |
59.00 |
5.64 |
5.64 |
5.64 |
1 |
1.82 |
3.64 |
8 |
11 |
|
A340–600 (TA, TF, LW) |
59.39 |
5.64 |
5.64 |
5.64 |
1 |
1.60 |
3.32 |
8 |
9 |
|
A380 (TA, TF, LW) |
70.40 |
7.78 |
8.41 |
7.14 |
|
1.50 |
3.91 |
5 |
11 |
|
Boeing 737 (TA, TF, LW) |
31.28 |
3.95 |
4.11 |
3.79 |
|
1.10 |
2.80 |
7 |
15 |
|
Boeing 747 (TA, TF, LW) |
68.63 |
7.30 |
8.10 |
6.50 |
|
1.31 |
3.31 |
5 |
11 |
|
Boeing 757 (TA, TF, LW) |
46.96 |
4.05 |
4.00 |
4.10 |
|
1.64 |
2.91 |
6 |
13 |
|
Boeing 767 (TA, TF, LW) |
47.24 |
5.03 |
5.03 |
5.03 |
1 |
1.17 |
2.67 |
7 |
15 |
|
Boeing 777 (TA, TF, LW) |
63.73 |
6.20 |
6.20 |
6.20 |
1 |
1.23 |
2.85 |
7 |
13 |
|
MD11 (TA, TF, LW) |
58.65 |
6.02 |
6.02 |
6.02 |
1 |
1.45 |
2.82 |
5 |
13 |
|
Tupolev 204 (TA, TF, LW) |
46.10 |
3.95 |
3.80 |
4.10 |
|
1.46 |
2.96 |
5 |
9 |
|
Fokker 100 (TA, TF, LW) |
32.50 |
3.30 |
3.05 |
3.49 |
|
1.42 |
3.42 |
2 |
10 |
|
Dornier 728 (TA, TF, LW) |
27.03 |
2.56 |
2.05 |
3.25 |
|
1.34 |
2.60 |
5 |
13 |
|
Dornier 328 (RA, TF, LW) |
20.92 |
2.42 |
2.425 |
2.415 |
|
1.27 |
2.64 |
5 |
10 |
|
Dash8 Q400 (RA, TP, HW) |
25.68 |
2.07 |
2.03 |
2.11 |
|
1.71 |
3.22 |
4 |
9 |
|
Bae RJ85 (RA, TP, HW) |
28.55 |
3.56 |
3.56 |
3.56 |
1 |
1.46 |
2.62 |
4 |
12 |
|
Skyvan (RA, TP, HW) |
12.22 |
square |
2.20 |
2.20 |
|
0.95 |
2.00 |
9 |
0 |
|
Cessna 560 (BJ, TF, LW) |
15.79 |
5.64 |
5.64 |
5.64 |
1 |
2.05 |
2.91 |
2 |
8 |
|
Learjet 31A (BJ, TF, LW) |
x |
5.64 |
1.63 |
1.63 |
|
2.17 |
3.64 |
2 |
5 |
|
Cessna 750 (BJ, TF, LW) |
21.00 |
1.80 |
1.80 |
1.80 |
1 |
2.00 |
3.00 |
7 |
15 |
|
Cessna 525 (BJ, TF, LW) |
14.00 |
1.60 |
1.60 |
1.60 |
1 |
2.00 |
2.56 |
7 |
13 |
|
Learjet 45 (BJ, TF, LW) |
|
5.64 |
1.75 |
1.72 |
|
1.91 |
2.86 |
8 |
4 |
|
Learjet 60 (BJ, TF, LW) |
17.02 |
3.96 |
1.96 |
1.96 |
1 |
1.91 |
2.82 |
2 |
5 |
|
CRJ 700 (RA, TF, LW) |
|
2.69 |
2.69 |
2.69 |
1 |
1.60 |
3.15 |
5 |
12 |
|
ERJ 140 (RA, TF, LW) |
26.58 |
|
|
|
|
2.00 |
2.89 |
3 |
14 |
|
ERJ 170 (RA, TF, LW) |
29.90 |
3.15 |
3.35 |
2.95 |
|
1.56 |
2.67 |
3 |
13 |
|
C17 (MT, TF, HW) |
49.50 |
6.85 |
6.85 |
6.85 |
1 |
0.85 |
3.41 |
10 |
12 |
|
C130 (MT, TF, HW) |
34.37 |
4.33 |
4.34 |
4.32 |
|
0.95 |
2.56 |
9 |
12 |
|
Notes: |
|
|
TA – Transport aircraft |
LW – Low wing |
H – Fuselage height |
RA – Regional aircraft |
HW – High wing |
W – Fuselage width |
BJ – Business jet |
L – Fuselage length |
Lf – Front-closure length |
MT – Military transport |
D – Fuselage diameter |
Lf – Aft-closure length |
TF – Turbofan |
|
UA – Upsweep angle, deg |
TP – Turboprop |
|
CA – Closure angle, deg |
fuselage to fit flat glasses on a curved fuselage body; flat surfaces permit wiper installation and are less costly to manufacture. Some small aircraft have curved windscreens that permit smooth fuselage mould lines.
The aft-end closure is shallower to minimize airflow separation when the boundary layer becomes thicker. All fuselages have some upsweep for aircraft rotational clearances at takeoff. The difference in shaping is minor and is a result of the designer’s choice. Designers must configure a satisfactory geometry with attention to all operation and structural requirements (e.g., pilot vision polar [see Section 4.7.4], pressure bulkhead positions, and various doors). Table 4.4 lists typical guidelines for the fuselage frontand aft-end closure ratios; the range represents current statistical values.