
диафрагмированные волноводные фильтры / a66f954d-d8c4-4dbc-ba70-4142774e9165
.pdf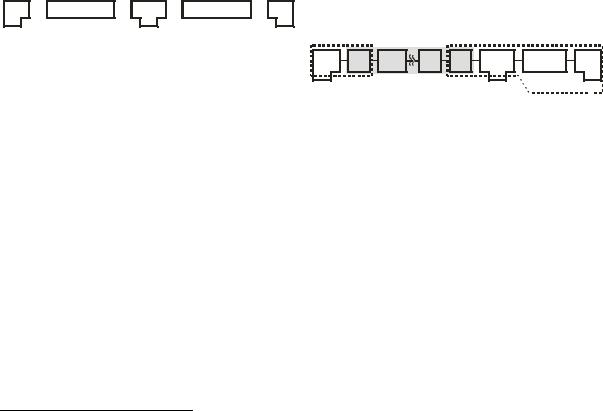
Synthesis of Wideband Waveguide Diplexers
G.Virone, R. Tascone, O. A. Peverini, G. Addamo and R. Orta1
Abstract − A design strategy for high-performance waveguide diplexers is presented in this paper. This technique directly provides the geometry of the two filters starting from the desired frequency response expressed in polynomial form. The dispersive mutual loading effect of the two filters and the various junctions as well as the losses and the multimodal interaction are kept into account. A diplexer with two 7-cavity filters in double-ridge waveguide with a -50 dB transition bandwidth of 1.4% and a return loss of 23 dB is reported.
1 INTRODUCTION
Modern telecommunication systems require several filtering structures to combine (separate) the various carriers into (coming from) a common port. Waveguide diplexers are still the most suitable solution when both high power handling capabilities and low losses are required.
A typical configuration is sketched in Fig. 1. Two pass-band filters (L and H) are connected to a T- junction in order to obtain a common port (Port 1). The other end of the filters is connected to proper waveguide transitions to provide the required orientation and size of Port 2 and 3.
|
|
Filter L |
2’ |
|
3’ |
Filter H |
|
|
|
|
|
|
|
|
|
||
Port 2 |
|
Port 1 |
|
|
Port 3 |
Figure 1: in-line waveguide diplexer scheme.
The common electrical requirements are a high transmission coefficient from Port 1 to Port 2 (Port 3), high attenuation from Port 1 to Port 3 (Port 2) and a low reflection coefficient at Port 2 (Port 3) in the lower (higher) frequency band. A low reflection coefficient at Port 1 for both frequency bands is also required.
A common diplexer design strategy usually starts with the synthesis of the two pass-band filters. Afterwards, the T-junction and the connection lengths are adjusted to obtain the above mentioned frequency behavior [1]. However, when broadband operative conditions are required, the mutual loading of the two filters and the T-junction has to be considered. This phenomenon has in fact a worsening effect on the reflection coefficient of the overall structure. Therefore, the geometry of the two filters has to be modified to compensate for the loading effect. In the literature, this task is generally performed by means of optimization algorithms applied to the overall structure [2].
A direct design strategy which naturally keeps the loading phenomenon into account is instead presented
in section 2. Roughly speaking, the first (last) discontinuity of the filter that has to be designed and the adjacent loading structure (i.e. the waveguide transitions or the T-junction with one port connected to the opposite filter) are treated as a single aggregate discontinuity. In this way, the synthesis procedure reported in [3] can be directly applied to obtain the geometry of the two loaded filters starting from the given specifications on the frequency behavior.
An E-plane diplexer in double-ridge waveguide is reported in section 3 as a design example.
2 AGGREGATE DISCONTINUITY
The diplexer equivalent transmission line circuit is reported in figure 2, where S (kL) are the scattering
matrices of the discontinuities of the filter L, S (H ) is
the scattering matrix of the whole filter H and S (TJ ) ,
S (T 2) |
and |
S (T 3) are the |
scattering |
matrices |
of the |
various junctions. |
|
|
|
||
Aggregate |
|
Aggregate |
|
||
Discontinuity |
|
Discontinuity |
|
||
S(T2) |
SN(L) |
SN-1(L) S2(L) |
S1(L) 2’ S(TJ)3’ |
S(H) |
S(T3) |
Port 2 |
|
Unloaded |
Port 1 |
Filter H |
Port 3 |
|
Filter L |
|
|||
|
|
|
|
|
Figure 2: equivalent transmission line circuit of the loaded filter L
The various circuit elements have been arranged to illustrate the design of the filter L in presence of the loading structures i.e. the waveguide transition on the left and the T-junction with port 3’ connected to the filter H and the corresponding waveguide transition on the right. An analogous circuit can be defined for the design of the filter H.
According to figure 2, the loading structure on the right and the first discontinuity of the unloaded filter are treated as a unique entity corresponding to the first discontinuity of the loaded filter. The same aggregation has to be done for the loading structure on the left and the last discontinuity of the filter L in order to define the last discontinuity of the loaded filter. This aggregation procedure is possible since the synthesis technique reported in [3] is based on a distributed parameter model and the discontinuities are described
1 Istituto di Elettronica ed Ingegneria dell’Informazione e delle Telecomunicazioni – Consiglio Nazionale delle Ricerche (IEIIT-CNR) c/o Politecnico di Torino, C.so Duca degli Abruzzi 24, 10129, Turin, Italy. Email: riccardo.tascone@polito.it, giuseppe.virone@polito.it.
978-1-4244-3386-5/09/$25.00 ©2009 IEEE
459

in terms of their scattering matrices. Such a synthesis technique directly yields the scattering parameters of the various filter discontinuities and the cavity lengths starting from the desired frequency behavior in polynomial form. Subsequently, the various geometrical parameters are determined using a look- up-table for the corresponding discontinuities.
The aggregate discontinuity is of course more dispersive than a simpler discontinuity (septum, iris, etc…). Nevertheless, its behavior can still be handled using the identification procedure reported in [3]. It has to be noted that multimodal interaction is also kept into account using this technique.
Since the mismatch of the loading structures on both sides of the unloaded filters is kept into account during the design, very simple transitions/junctions (without complex matching elements) can be adopted in order to simplify the diplexer manufacturing.
It has to be pointed out that the synthesis of the loaded filter is not independent of the geometry of the loading filter. However, since the loading filter exhibits a stopband behavior at the design frequencies of the loaded filter, its loading effect only slightly depends on subsequent design refinements. Therefore, the synthesis procedure can be applied alternatively on the two filters and only few iterations are required to reach convergence.
been obtained at ports 2 and 3. The two transmission coefficients reach an attenuation higher than 50 dB in the 1.4% central transition bandwidth. The attenuation is also higher than 50 dB at the lower and upper stopband limits (0.945 f0 and 1.055 f0). The computed insertion loss is about 0.6 dB at the center frequencies and below 1.3 dB at the band limits (assumed surface resistivity 3 μΩcm).
0 |
|
|
|
|
|
|
|
|
|
|
|
|
S11 |
-10 |
|
|
|
|
|
S21 |
|
|
|
|
|
|
S31 |
-20 |
|
|
|
|
|
|
-30 |
|
|
|
|
|
|
dB |
|
|
|
|
|
|
-40 |
|
|
|
|
|
|
-50 |
|
|
|
|
|
|
-60 |
|
|
|
|
|
|
-70 |
0.96 |
0.98 |
1 |
1.02 |
1.04 |
1.06 |
0.94 |
||||||
|
|
Normalized Frequency ( f / f0 ) |
|
|
Figure 3: scattering parameters of the designed diplexer.
3 DOUBLE-RIDGE WAVEGUIDE DIPLEXER
An in-line waveguide diplexer has been designed according to the presented procedure. The two 7-cavity filters have been implemented using E-plane septum discontinuities in the double-ridge waveguide [4]. This configuration is in fact more compact than the common E-plane one in rectangular waveguide because the resonators and the septa are shorter owing to the lower cut-off frequency of the ridge waveguide and the higher reflection level of the ridge-septum- ridge discontinuities, respectively. A proprietary simulation code has been used for the full-wave analysis [5].
The proposed structure can be manufactured using the typical clam-shell technique where the two filters share the same waveguide housing. Two L-junctions are placed at the two ends of the diplexer to provide the required waveguide port rotation. Both the L- junctions and the central T-junction are mitered. Their geometry can be rounded to allow a cost-effective manufacturing (milling). Both the double-ridge waveguide sections (resonators) and the septa can be realized on the same metal sheet.
Fig. 3 shows the obtained normalized frequency response ( f / f0 ). Reflection levels of -23 dB and -26 dB have been obtained at the common port 1 (see Fig. 1 for the port numbering) for the lower (0.963-0.993 f0) and higher (1.007-1.037 f0) passbands, respectively. Similar levels (not shown) have
References
[1]R. Vahldieck, B. Varailhon de la Filolie, “Computed Aided Design of Parallel-connected Millimeter-wave Diplexers/Multiplexers”, IEEE MTT-S Digest 1988, pp. 435-438.
[2]J. Dittloff, F. Arndt, “Rigorous Field Theory of Millimeter-Wave E-plane Integrated Circuit Multiplexers”, IEEE T-MTT Vol. 37, n. 2, Feb 1989, pp. 340-350.
[3]R. Tascone, P. Savi, D. Trinchero, and R. Orta, “Scattering matrix approach for the design of microwave filters,” IEEE T-MTT., vol. 48, no. 3, Mar. 2000 pp. 423–430.
[4]G. Goussetis and D. Budimir, “E-Plane Double Ridge Waveguide Filters and Diplexers for Communication Systems”, European Microwave Conference, 2001, 31st Oct. 2001, Page(s):1-4, DOI 10.1109 /EUMA.2001.339059
[5]O. A. Peverini, R. Tascone, M. Baralis, G. Virone, D. Trinchero and R. Orta, “ReducedOrder Optimized Mode Matching CAD of Microwave Waveguide Components”, on IEEE Transactions on Microwave Theory and Techniques, Vol. 52, Issue 1, Jan. 2004, pp. 311318.
460