
диафрагмированные волноводные фильтры / e4868852-11c1-4165-8d4d-cfd8ee1c86d8
.pdf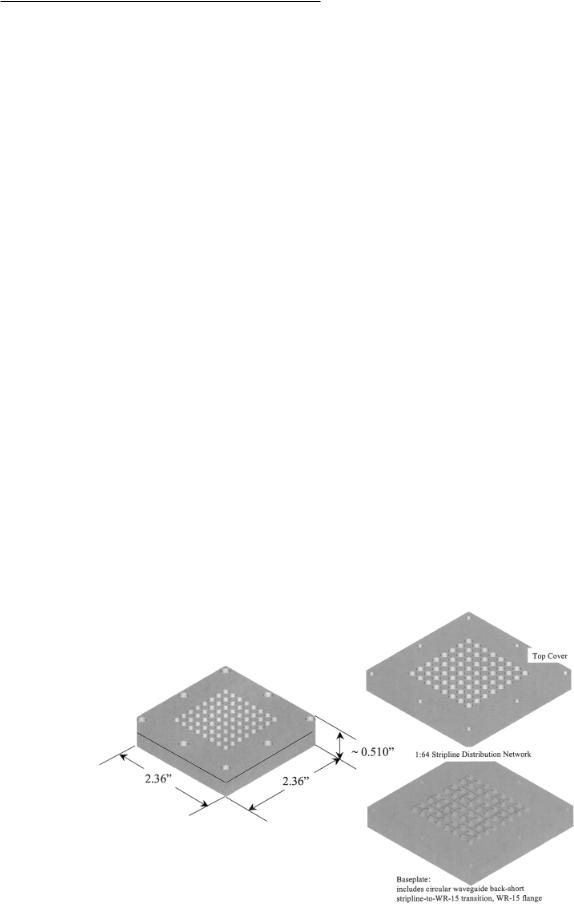
WIDE-BAND, LOW-PROFILE MILLIMETER-WAVE ANTENNA ARRAY
Julio Navarro
Omni-Patch Designs, Inc.
11207 SE 253rd Place
Kent, Washington 98032
Received 10 January 2002
ABSTRACT: A low-profile 60-GHz antenna array has been designed, built, and tested. The design uses a circular waveguide radiator for bandwidth, a stripline distribution network for minimum thickness and flexibility, and a waveguide input for interfacing to other components. Boresight gain variations of less than 1.5 dB were demonstrated for several units over 57– 63 GHz. © 2002 Wiley Periodicals, Inc. Microwave Opt Technol Lett 34: 253–255, 2002; Published online in Wiley InterScience (www.interscience.wiley.com). DOI 10.1002/mop. 10430
Key words: millimeter wave; antenna array; low-profile antenna
1. INTRODUCTION
Millimeter-wave components have been dominated primarily by waveguide due to efficiency and power-handling considerations. As radiators, waveguides also provide wide percentage bandwidths. Planar components provide manufacturing advantages, repeatability, and lower costs at the expense of efficiency. Planar distribution networks are more lossy than their waveguide counterparts but are more compact, doubly conformal, and can distribute RF energy to very small regions of a circuit.
In the design of antenna arrays, waveguide feed networks are often limited by very tight spacings. Smaller element spacings often force waveguide feed networks to be series feeds. Series-fed arrays are inherently narrow band. Any application with more than a few percent of instantaneous bandwidth requires the use of a corporate feed network.
This submission describes the design, fabrication, and testing of a low-profile millimeter-wave array that exhibits reasonable efficiency, pattern integrity, and boresight gain variations from 57
to 63 GHz. Areas that limit the current design in bandwidth, efficiency, and cost are addressed along with possible solutions and improvements. The low-profile design can be used as is or as a sub-array in a larger antenna or even as a feed approach for phased-array modules.
2. ARRAY DESIGN
The objective of the design of this array was to develop a conformal wideband planar array. The design criteria included reducing the boresight gain variation to less than 1.5 dB over a 10% bandwidth. Several microstrip configurations have advantages in simplicity and cost but often lack the bandwidth required to maintain these requirements. A simple pyramidal horn has ideal boresight gain variation over a large bandwidth at the expense of depth. This design integrates a circular waveguide radiator with a planar distribution network fed by rectangular waveguide. It provides a good balance between pattern performance, efficiency, conformability, and bandwidth.
Figure 1 shows the dimensions of the 64 element strip-line–fed circular waveguide array along with an exploded view. Half of the overall 0.510-in. thickness is due to mechanical considerations. The top cover as shown in Figure 1 is approximately 0.200 in. It contains a rectangular array of equally spaced 64 circular holes for the radiators. Circular radiators were chosen over rectangular waveguide because of ease in drilling. The remaining holes are for alignment during assembly and mounting. There are two 0.005- in.-thick Rogers 5880 substrates with 0.5-oz copper where the RF distribution network resides. The strip-line distribution network is sandwiched between the top cover and base plate. The drilled circular radiators on the cover need to lined up with the distribution layer and base plate. The bottom base includes a small length of circular waveguide back short for the transition to the radiator. The base also has the strip-line–rectangular-waveguide transition. The input to the array is a WR-15 input on the back of the base plate.
The design of a circular waveguide radiator is well known. Single-mode operation can be maintained over a 33% bandwidth. A 5% margin above cutoff and before the onset of the next higher mode plus some matching considerations makes this radiator suit-
Figure 1 Assembled and exploded view of 64-element array
MICROWAVE AND OPTICAL TECHNOLOGY LETTERS / Vol. 34, No. 4, August 20 2002 |
253 |

Figure 2 Electrical schematic for 64-element array
able for use over a 20% bandwidth. Circular waveguides can be fed with an h-plane loop, e-plane probe, or a slot aperture. The orientation of the waveguide/distribution network made the e-plane probe ideal as a transition from the distribution network to the radiator. A slot aperture feed could also be used. In order to save on costs, the stripline network is not laminated as a multilayer printed wiring board (PWB). Mode-suppression vias are also not included in this distribution. Instead, the two substrates are lined up and pressed together with the use of the base plate and cover. The inclusion of vias would allow the use of a thicker strip-line layer that will reduce losses in the distribution and increase gain. Simple reactive 3-dB splitters were used throughout the 64-ele- ment corporate feed network. The strip-line–fed circular waveguide was simulated and the results were used as loads in the strip-line distribution network. The simulations show good performance from 55– 65 GHz given the assumptions in the loads. Alignment errors between the strip-line probes and circular waveguides degrades the input match at each radiator. The distribution does not have isolation resistors; therefore, mismatch errors
at each radiator create standing waves along the distribution network.
One end of a coaxial probe was soldered to the input of the strip-line distribution network. The other end was used to transition to a rectangular waveguide with the use of an e-plane probe and back-short. There is a 90 degree E-plane bend included in the baseplate which allows a WR-15 waveguide output parallel to the plane of the antenna array. Individually, each of the transitions and E-plane bend can be designed for more than 20% bandwidths. However, reflections and interactions between each of the transitions reduce the efficiency and overall bandwidth performance of the array. Figure 2 shows a schematic representation and a top see-through look of the 64-element array.
3. MEASURED RESULTS
Several 64-element arrays were fabricated, assembled, and tested. An HP-8510B network analyzer along with a 5 multiplier, dual directional couplers, and harmonic mixers was used to measure the
Figure 3 E-plane radiation contour and patterns from 55 to 65 GHz
254 MICROWAVE AND OPTICAL TECHNOLOGY LETTERS / Vol. 34, No. 4, August 20 2002

Figure 4 H-plane radiation contour and patterns from 55 to 65 GHz
return loss and array gain. Figure 3 shows E-plane radiation patterns and contour plot from 55 to 65 GHz. Similarly, Figure 4 shows radiation patterns and contour for the H plane. Figure 5 shows the boresight gain versus frequency for the 64-element array.
Three such arrays were built, the best results are shown in the figures. The distribution was designed to feed an equal amplitude antenna array. Near side lobes varied a couple of decibels around13 dB throughout the 55– 65-GHz bandwidth. Boresight halfpower beam width remains nearly constant throughout the measured bandwidth. Increase in side-lobe level occurs at a couple of frequency points, which may be due to a variety of reasons, including mismatch interactions, coupling within distribution networks, and/or measurement setup. The boresight gain variation is within a couple of decibels throughout most of the bandwidth. The overall efficiency of the antenna array varies between 30 and 40%. Current research efforts are trying to assess how the losses are distributed throughout the array. The loss mechanisms include the radiator, circular waveguide–strip-line transition, distribution split-
ters, and line losses, strip-line–rectangular waveguide transition, and a WR-15 E-plane 90° bend.
4. CONCLUSION
A low-profile, wide-band antenna array concept has been designed, built, and tested. The 64-element array uses rectangular waveguide, coax, strip line and circular waveguide. The radiation patterns remain fairly consistent throughout the measured bandwidth, and overall efficiency is reasonable at around 35%. The boresight gain variation is better than expected and efforts to reproduce and improve these results with the other two units are under way.
© 2002 Wiley Periodicals, Inc.
A NOVEL SUPERCONDUCTING CPW
SLOW-WAVE BANDPASS FILTER
Jiafeng Zhou, Dungshing Hung, Michael J. Lancaster,
Hieng Tiong Su, and Xuming Xiong
School of Electronic, Electrical and Computer Engineering
The University of Birmingham
Edgbaston, Birmingham B15 2TT, UK
|
Received 7 February 2001 |
|
ABSTRACT: A novel slow-wave half-wavelength coplanar waveguide |
|
(CPW) resonator using high-Tc superconducting (HTS) films has been |
|
studied and used in a new filter topology. The slow-wave effect is |
|
achieved by the capacitively loaded structure with a symmetrical inter- |
|
digital line loaded on both sides of the central line. The velocity reduc- |
|
tion (Vp/c0) of the resonator is about 0.050. A three-pole Butterworth |
|
bandpass filter has been designed and fabricated with the use of the |
|
resonator. © 2002 Wiley Periodicals, Inc. Microwave Opt Technol |
|
Lett 34: 255–259, 2002; Published online in Wiley InterScience (www. |
|
interscience.wiley.com). DOI 10.1002/mop.10431 |
Figure 5 Boresight gain variations from 55 to 65 GHz |
Key words: bandpass filter; slow wave; interdigital gap; HTS; CPW |
MICROWAVE AND OPTICAL TECHNOLOGY LETTERS / Vol. 34, No. 4, August 20 2002 |
255 |