
диафрагмированные волноводные фильтры / de7ce2de-f8a0-4c63-92e7-fa8e5e9928f7
.pdf
Received: 25 October 2018 Revised: 29 January 2019
Accepted: 15 March 2019
DOI: 10.1002/mmce.21773
R E S E A R C H A R T I C L E
Design and performance comparison of a novel high Q coaxial resonator filter and compact waveguide filter for millimeter wave payload applications
Vineet K. Dad1,2 | Sanjeev Gupta1
1RF and Microwave Department, DA-IICT,
Gandhinagar, Gujarat, India
2Payload Passive Component Divison,
Engr., SAC, Ahmedabad, Gujarat, India
Correspondence
Vineet K. Dad, DA-IICT, Gandhinagar,
Gujarat, India.
Email: vineet.dad@gmail.com, 201321004@daiict.ac.in
Abstract
A novel high-performance high Q filter with ultra-wide spurious-free stopband and thermally stable performance is introduced using higher order mode of the coaxial resonator. The 7-pole Chebyshev and a 5-pole cross-coupled filter are designed at Ka-band to validate the concept. A compact cross-coupled waveguide filter is introduced with a different approach to realize cross-coupling makes the filter compact. The coaxial resonator and waveguide resonator filter have the same configuration, and their performances are compared at ambient and over the temperature. The high power analysis is done for both these filters to find their power handling capability. The high power performance of the coaxial resonator filter is superior, and it can handle 45.525 kW peak power. This filter is suitable to be used as pre-select filter in millimeter wave payloads as well as for high power filtering applications.
K E Y W O R D S
coaxial resonator, cross-coupled filter, high power, higher order mode, ka-band, millimeter-wave,
multipaction, waveguide filter
1 | INTRODUCTION
The advancement in the communication payload technology demands high-performance filters with the smaller footprint, lesser mass, and good power handling capability at highfrequency bands. The low insertion loss and spurious-free band filters are deployed at the front-end of payload to reject out-of-band interference before down conversion. These filters are either all pole or pseudo-elliptic which provide skirt selectivity and improved in-band performance for efficient utilization of spectral power. The cross-coupling is realized by creating a discontinuity between the nonadjacent resonators which bring transmission zeros from infinity to finite location in the complex plane, results in skirt selectivity and improved group delay performance. The resonators used for the realization of low loss filters for millimeter-wave payloads should have the high-quality factor, wide spurious free-range, and stable RF response over temperature. The
three-dimensional (3D) resonators like cavity resonator, coaxial resonator, dielectric resonators possess high Q due to its large ratio of volume to surface area. The use of cavity resonator based filters are popular in millimeter-wave payload due to its high Q, very-wide spurious-free range, fairly good size, predictable thermal performance, ease of integration with other subsystem and high power handling capability. The planar and micromachined structures are the other technologies used for millimeter wave filter realization,1,2 but they have low Q. The dominant TEM mode of coaxial resonators have high Q, small size and stable thermal performance till X-band but it will degrade with increase in the frequency. The filters developed with the TEM mode coaxial resonator are direct-coupled or cross-coupled, and in each form, the emphasis is on performance improvement and size reduction.3–6
The higher order modes of resonators have high Q, but they have a poorer spurious free range. The higher-order
Int J RF Microw Comput Aided Eng. 2019;e21773. |
wileyonlinelibrary.com/journal/mmce |
© 2019 Wiley Periodicals, Inc. |
1 of 10 |
https://doi.org/10.1002/mmce.21773

2 of 10 |
|
|
|
|
DAD AND GUPTA |
|
|
|
|
||
|
|
|
|
|
|
|
|
|
|
|
|
|
|
|
|
|
|
TE221 mode of the circular cavity is used to develop filter at 19.9GHz. This cavity mode has very high Q but an inferior spurious-free window, which limits its use for narrowband channel filters of the input multiplexer.7 The higher-order modes of the coaxial resonators are using for gyrotron application.8 Recently, the authors have developed a Ka-band filter for the first time with the higher order TM012 mode of the coaxial resonator.9 This mode has Q better than 3000 at 30.5GHz, very-wide spurious free window and excellent thermal stability, makes it to be used as an input filter for millimeter wave payloads.
This article will discuss in detail about the design of TM012 mode coaxial resonator based filter, which includes design chart provide irises dimension for the coupling values, concept validation with the example of a direct-coupled 7-pole Chebyshev and a cross-coupled 5-pole cross-coupled filter and compares the performance of the proposed filter with the existing competing technology waveguide resonator at millimeter-wave band. The first section of this article presents the design of TM012 mode coaxial resonator filter with the example of 7-pole Chebyshev and 5-pole cross-coupled filter. The second section introduces the design and measured results of identical configuration cross-coupled rectangular waveguide filter where a different approach for crosscoupling realization is used, makes filter compact.
In each section, the fabricated filter measured results presents after the design. The last section discusses the high power analysis of 5-pole cross-coupled filter made from these two different technologies, followed by their performance comparison.
2 | HIGHER ORDER MODE
COAXIAL RESONATOR FILTER
The coaxial resonator is a metallic post placed in the cavity where the shape of both cavity and post may be either rectangular or circular. The commercially available eigenmode solver is used to calculate the mode frequencies of first six modes of the coaxial resonator assuming silver plating on it. Figure 1A shows the variation of modes frequency in GHz against cavity diameter for a fixed cavity height and on the secondary axis variation of Q-factor for TM012 mode9 in the mode chart. The first six modes of cylindrical shape coaxial resonator are dominant TEM, dual mode TE111, TM012, and dual mode TE112. The increase in the cavity height increases the quality factor of TM012 mode with an insignificant impact on the spurious-free window.
Similarly, the mode chart is plotted for the first six modes of similar dimension cylindrical cavity as shown in Figure 1B. The dominant mode is dual-modeTE111, and its simulated Q-factor is better than 6500. The next higher mode is TM011 has Q better than 6900. Then the next higher
modes are TM012 and TE112. The spurious-free window between dominant mode and the next higher mode is 3GHz, which reduces to 1.5GHz as diameter increases from 6.8 to 7.8 mm, respectively. The best spurious-free window is 4GHz at one end and 5GHz on the other end for TM012 mode, but the filter design with this mode have poor wide stopband due to spurious modes.
2.1 | TM012 mode
The TM012 is the fourth mode in the mode chart, and its electric and magnetic field patterns are analogous to the TM01 mode of the cavity resonator. These field patterns are shown in Figure 2 where there is one variation of the magnetic field along azimuth, two variations along Z-axis, and zero variation along the radial direction. The maximum field available near the top of the cavity is sufficient to realize narrow as well as the wide-passband bandpass filter. The quality factor of more than 4800 is simulated considering silver metal boundary for a single resonator. The dimension of the cavity and post are 7.2 mm diameter and 9 mm height and 3 mm diameter and 4 mm height, respectively. The spurious-free window of 7.1 and 6 GHz, below and above the resonant frequency is sufficient to realize wideband filters.
2.2 | Filter design
The TM012 mode of the coaxial resonator is used to design two different filters for the specification given in the Table 1. These specifications can be met using resonator Q-
factor of 2800. |
The first filter is an inline 7-pole Chebyshev |
|
filter designed |
for 0.01 dB passband |
ripple. Its coupling |
values are |
MS1 = M7L = 1.1129, |
M12 = M67 = 0.9416, |
M23 = M56 = 0.6382 and M34 = M45 = 0.5900 where S, L are source and load and 1 to 7 are resonators. In the design of the cross-coupled filter, two transmission zeros are at ±1.45j in the synthesis,10 and Table 2 gives synthesized coupling matrix for a 5-pole filter with two transmission zeros, that is, 5-2-0 filter, where S and L are source and load and 1 to 5 are resonators. The cross-coupling is between nonadjacent resonator 1 and 4.
In both of these filters, all the inter-resonator couplings are realized using inductive irises placed at the top of the cavity where the field is maximum for coupling. The crosscoupling is realized using a non-resonating post, and it is between two nonadjacent resonators11 where the length of post dictates nature of cross-coupling.
Figures 3 and 4 show inter-resonator coupling bandwidths, and is calculated by varying the iris height and iris width between the resonators. The coupling bandwidth is the separation between the eigen frequencies of the two identical resonators separated by an iris. It is calculated using

DAD AND GUPTA |
|
|
|
|
3 of 10 |
|
|
|
|
||
|
|
|
|
|
|
|
|
|
|
|
|
|
|
|
|
|
|
F I G U R E 1 A, Mode chart of coaxial resonator. B, Mode chart of
cylindrical cavity resonator
eigenmode solver of the 3D EM solver. The normalization of coupling bandwidth with passband bandwidth gives the required coupling values (κ) corresponds to the irises dimensions, which realize inter-resonator couplings.
κ= Coupling Bandwidth Passband Bandwidth
It is found from Figure 3 that the maximum coupling achieved when iris height is half of the cavity height. The trend is the same as TEM mode of coaxial resonator where coupling varies from minimum to maximum and then the
F I G U R E 2 Field patterns of coaxial resonator
minimum with variation in the iris height from top to bottom of the cavity.
The coupling bandwidth increases with increase in iris width. When the iris height is half of the cavity height, and iris width opening is maximum, the coupling bandwidth will be maximum. The spurious mode is also varying with iris width and iris height. The iris width should be increased instead of increasing iris height to keep spurious away from far passband. Moreover, up to 10%, fractional bandwidth filter can be realized at millimeter wave using this concept.
The coupling bandwidth increase with increases in the iris width as shown in Figure 4A. The non-resonating post coupling bandwidth variation against length for 3 mm iris height and 3.5 mm iris width is shown in Figure 4B. The coupling nature is negative when the post length is more than 1.62 mm. Figures 3 and 4 gives initial irises dimension of inter-resonator coupling which corresponds to the coupling matrix value. These irises and resonator post dimensions are optimized using the calculated reflection group delay values9.
The frequency drift of the filter calculated by an approximate relation given by
δf = α*f0*δT
where, δf is the frequency drift over the temperature, f0 is the center frequency, δT is the temperature gradient in C, and α is the coefficient of thermal expansion for metal in ppm/ C. The filter is fabricated in Aluminum (α = 24 ppm/0C). One side frequency drift is 29.04 MHz for the filter operating in the temperature range of 25 C to 65 C.
In 7-pole Chebyshev filter, the irises height is 4.5 mm for all inter-resonator coupling and the irises width are varying according to Figure 4A for the synthesized coupling values. The 7-pole Chebyshev filter simulated using reflection group delay values13 in CST Microwave Studio.12 Figure 5 shows this filter, which is fabricated with aluminum and is silver plated to minimize loss. The calibration is done on 2-port VNA using WR-28 waveguide calibration kit and the fabricated filter test with this set up. Its simulated and measured response has shown in Figure 6. This filter is meeting all the
specifications |
of Table 1 except insertion |
loss, which is |
T A B L E 1 |
Typical specification of Ka-band pre-select filter |
|
|
|
|
Specification |
|
Values |
|
|
|
Frequency band |
30.0-30.5 GHz |
|
|
|
|
Insertion loss |
|
< 0.5 dB |
Bandpass flatness over BW |
< 0.3 dB |
|
|
|
|
Rejection at CF ± 450 MHz |
>20 dB |
|
Rejection at 20 GHz |
>90 dB |
|
|
|
|
Return loss |
|
>20 dB |
Input and output interface |
WR-28 |
|
|
|
|

4 of 10 |
|
|
|
|
DAD AND GUPTA |
|
|
|
|
||
|
|
|
|
|
|
|
|
|
|
|
|
|
|
|
|
|
|
T A B L E 2 |
Synthesis coupling matrix for 5-2-0 filter |
|
|
|
|
|
|
|
|
|
|
|
|
|
|
|
|
|
S |
1 |
2 |
3 |
4 |
|
5 |
L |
|
|
|
|
|
|
|
|
|
S |
0 |
1.1226 |
0 |
0 |
0 |
0 |
0 |
|
|
|
|
|
|
−0.3270 |
|
|
|
1 |
1.1226 |
0 |
0.9029 |
0 |
|
0 |
0 |
|
2 |
0 |
0.9029 |
0 |
0.8427 |
0 |
0 |
0 |
|
|
|
|
|
|
|
|
|
|
3 |
0 |
0 |
0.8427 |
0 |
0.5949 |
|
0 |
0 |
4 |
0 |
−0.3270 |
0 |
0.5949 |
0 |
0.9603 |
0 |
|
|
|
|
|
|
|
|
|
|
5 |
0 |
0 |
0 |
0 |
0.9603 |
|
0 |
1.2266 |
L |
0 |
0 |
0 |
0 |
0 |
1.1226 |
0 |
|
|
|
|
|
|
|
|
|
|
0.78 dB. This high loss is due to improper contact between the box and top cover.
The 5-2-0 filter layout is shown in Figure 7A where inter-resonator irises height is fixed to 4.5 mm and Figure 4A coupling bandwidth normalized with 500 MHz passband bandwidth for the coupling values. The interresonator irises width are 3.41, 3.37, 3.16, and 3.45 mm. The cross-iris height and width are fixed to 3 and 3.5 mm, respectively, but non-resonating post length is varying according to Figure 4B for the cross-coupling value of −0.3270. The 5-2-0 filter CAD model with these initial irises dimension is simulated by the reflection group delay method13 in the CST Microwave Studio. The filter is designed slightly higher than center frequency to take care of fabrication tolerances The Figure 7B shows the filter is fabricated in two parts with aluminum and is silver plated. This filter has tested on a 2-port VNA with WR-28 waveguide calibration kit. Figure 8 shows its wideband EM simulated response and measured response. This filter is meeting all the specifications of Table 1. The spikes around 26 GHz in the filter response show signature of TE11 mode as confirmed by mode chart, but this is a noninteracting mode and has rejection level below 58 dB. Therefore, it does not have any impact on out of band performance of the filter. The selection of tuning screws at millimeter-wave is critical due to contact between the screw and the tap hole, which plays a
dominant role in determining the performance of the fabricated filter. The measured response shows close agreement with the simulated response for both types of coaxial resonator filters.
3 | COMPACT CROSS-COUPLED WAVEGUIDE FILTER
The cross-coupling creates in the filter due to the destructive interference between the direct mainline path and nonadjacent cross-coupled resonator path.14 It is positive or negative depending on the type of the field coupled. The various approaches have shown till now with the folded
|
F I G U R E 4 A, Variation of coupling bandwidth and TE112 |
|
(spurious) mode resonance frequency with variation in iris width. B, |
F I G U R E 3 Variation of coupling bandwidth and TE112 |
Variation of coupling bandwidth with variation in the length of non- |
(spurious) mode resonance frequency with variation in iris height |
resonating post |

DAD AND GUPTA |
|
|
|
|
5 of 10 |
|
|
|
|
||
|
|
|
|
|
|
|
|
|
|
|
|
|
|
|
|
|
|
F I G U R E 5 7-Pole Chebyshev TM012 mode coaxial resonator filter
waveguide filters to realize the cross-coupling.15–20 The circular aperture is made in the H-plane of the folded waveguide resonator to generate the cross-coupling but there is difficulty in tuning the cross-coupling, and it is sensitive to fabrication tolerance which matters a lot at the millimeterwave band.15 The other popular approach is to use an overmoded cavity16,17 to generate transmission zeros. This approach enables the filter to handle very high power at the expense of increased filter size due to the large size overmoded cavity. Moreover, this approach is suitable to the narrow band filter design. The combination of a non-resonating TE10 mode with TM110 mode is used to realize crosscoupling make filter compact but at the expense of poor isolation in the lower stopband.18 This approach is inappropriate for the pre-select filter realization which offers high isolation to the transmit band. In ultra-compact crosscoupled waveguide filter, a metal strip is inserted in E-Plane of waveguide to generate transmission zeros.19 This approach is sensitive to fabrication tolerance due to the fixed dimensions of the thin strip. Moreover, the filter response is asymmetric due to the imbalance between side lobes. The capacitive irises increase the overall filter size and are better
F I G U R E 6 Simulated and measured response of 7-Pole
Chebyshev TM012 mode coaxial resonator filter
suited for very-wideband filter due to the aperture of the capacitive iris.20,21
Here we have used the different approach for crosscoupling realization where the non-resonating post has placed between nonadjacent resonators. The length of the post governs the nature of coupling. The filter has designed for the specification of Table 1 with 5-pole and two transmission zeros. The synthesized coupling matrix for the 5-2-0 filter has given in Table 2. The dominant TE101 mode of rectangular waveguide resonator used for filter design. This mode has Q-factor of better than 2800 at 30.5GHz and verywide spurious-free window. This non-resonating post is tunable which relaxes fabrication tolerances, and use of post does not add any additional cavity to the filter. Hence, it makes the filter compact. The inter-resonator coupling is realized using inductive irises, and the variation of coupling with iris width variation for fixed iris depth of 3.5 mm as shown in Figure 9. The inter-resonator coupling increases as the iris width aperture between the cavities increases.
The non-resonant post depth variation for fixed iris width aperture of 4 mm and iris depth of 3 mm shown in Figure 10. The coupling increases as the penetration of post increase to a maximum value and then it starts decreases drastically after inflection point.
The inflection point distinguishes between the nature of coupling as this post can realize both positive and negative coupling. Figure 10 shows that the inflection is at 2.16 mm. The coupling is positive before the inflection point and negative coupling after that. The post is placed normal to the magnetic field plane for realizing cross-coupling.
The filter is fabricated using aluminum material and is silver plated to reduce losses. The fabricated 5-2-0 waveguide filter shown in Figure 11. The M1.6 stainless steel (SS) screws with hexagonal locking nuts are used to tune the resonator center frequency, inter-resonator coupling, and cross-coupling. The filter is characterized using the WR-28 waveguide calibration kit on 2-port VNA, and Figures 12 and 13 show its measured responses.
The measured value of insertion loss is 0.45 dB, and the bandpass flatness is 0.25 dB. The far band rejection is better than 85 dB. There is a close match between the simulated response and the measured filter response. There is a minor imbalance between the side-lobe, and it is due to the spurious coupling between nonadjacent resonators. The filter mass is 26 g and footprint is 1200 mm2.
4 | HIGH POWER ANALYSIS
Multipaction is a high power RF vacuum breakdown phenomenon where there is a resonant growth of free electron space charge due to secondary electron emission.10,22 The high power handling capability of filters are established by

6 of 10 |
|
|
|
|
DAD AND GUPTA |
|
|
|
|
||
|
|
|
|
|
|
|
|
|
|
|
|
|
|
|
|
|
|
F I G U R E 7 A, 5-2-0 TM012 mode coaxial resonator filter layout. B, 5-2-0 TM012 mode coaxial resonator filter
the multipaction margin which is usually 6 dB for the filters used in space applications.21 The multipaction margin calculated and validated by design or by the multipaction test set up23. The design margin provides good accuracy when there is a limitation of the test setup. The steps to calculate multipaction margin are:
Step 1: Identify the critical gaps in the design. These are the locations where electric field intensity is maximum. These may or may not be the minimum gap.
Step 2: Calculate the threshold voltage (Vth) with avail-
able voltage breakdown curves.24–26 The threshold voltage for silver plated parallel plate geometry is given by24,27
Vth = 63*f*d
where f is the frequency in GHz and d is the critical gap in mm.
Step 3: Calculate normalized voltage (Vn). Evaluate the line integral of electric field in the commercial EM Solvers for 1 W power and is scale for operating power. The operating power corresponds voltage (V).
F I G U R E 8 Simulated and measured wideband response of 5-2-0 |
F I G U R E 9 Variation of coupling bandwidth with variation in iris |
TM012 mode coaxial resonator filter |
width aperture |

DAD AND GUPTA |
|
|
|
|
7 of 10 |
|
|
|
|
||
|
|
|
|
|
|
|
|
|
|
|
|
|
|
|
|
|
|
F I G U R E 1 0 Variation of coupling bandwidth with variation in
iris width aperture
F I G U R E 1 1 Fabricated 5-2-0 waveguide filter
Þ
Vn= E.dl
where E is the electric field in V/m.
Step 4: The multipaction margin “M” in dB is given by
M = 20*log V=Vth
The other method is to use the commercially available tools like SPARK 3D, PIC solver or custom-made codes.
F I G U R E 1 3 Simulated and measured wideband response of
5-2-0 waveguide filter
F I G U R E 1 4 Multipaction analysis of the proposed 5-2-0 filter
The high power analysis is carried out with the software28 for the 5-2-0 coaxial resonator and 5-2-0 waveguide filter, and observed that the peak power handling capacity of the proposed coaxial resonator filter is 45.525 kW as compared to the waveguide filter which is 11.75 kW only shown in Figures 14 and 15, respectively.
F I G U R E 1 2 |
Simulated and measured response of 5-2-0 |
waveguide filter |
F I G U R E 1 5 Multipaction analysis of the 5-2-0 waveguide filter |

8 of 10 |
|
|
|
|
DAD AND GUPTA |
|
|
|
|
||
|
|
|
|
|
|
|
|
|
|
|
|
|
|
|
|
|
|
F I G U R E 1 6 Thermal performance of the proposed 5-2-0 coaxial
resonator filter and 5-2-0 waveguide filter
The critical gap between the coaxial resonator post and the top cover is close to the parallel plate geometry, and the multipaction margin has calculated for the same. The critical gap is 3.825 mm, and the threshold voltage corresponds to it is 7289 V. The normalized voltage 143.02 V which is 15 000.8 V for 11.38 kW power. Hence, the calculated multipaction margin 6.26 dB corresponds to 45.525KW threshold power which validates the result of SPARK 3D with design margin.
The gap between the non-resonant post and iris between resonator-1 and 4 is minimum for both the filters. In the case of the proposed filter critical gap is between the coaxial resonator post-2,3 and its top cover. This gap is 15 times more than the minimum. However, in the case of waveguide filter, the minimum and critical gap are the same. Therefore, the proposed filter power handling capability is four times more than the waveguide filter. Moreover, the non-resonating post used for cross-coupling realization limits power handling capability of the waveguide filter. The waveguide filter realized by this approach can handle moderate power. The average power handling is 6 dB below the multipaction
T A B L E 3 Performance comparison of proposed filter and waveguide filter
|
|
Proposed |
Waveguide |
Specification |
Values |
filter |
filter |
|
|
|
|
mCentre frequency |
30.25 GHz |
30.25 GHz |
30.25 GHz |
(CF) |
|
|
|
|
|
|
|
Bandwidth (BW) |
500 MHz |
500 MHz |
500 MHz |
Insertion loss |
< 0.5 dB |
0.35 dB |
0.45 dB |
|
|
|
|
Bandpass flatness |
< 0.3 dB |
0.18 dB |
0.25 dB |
over BW |
|
|
|
Rejection at CF |
>20 dB |
38.46 dB |
29 dB |
± 450 MHz |
|
|
|
|
|
|
|
Rejection at 20 GHz |
>90 dB |
>90 dB |
85 dB |
Return loss |
>20 dB |
>25 |
>24 dB |
|
|
|
|
Input and output |
WR-28 |
WR-28 |
WR-28 |
interface |
|
|
|
Frequency drift |
… |
27 MHz |
29 MHz |
|
|
(22.31 ppm/ C) |
(24 ppm/ C) |
Mass |
… |
34 g |
26 g |
Foot print |
… |
1451mm2 |
1199 mm2 |
Power handling |
… |
11.38KW |
2.93KW |
capability |
|
|
|
|
|
|
|
threshold. Therefore, the proposed filter and the waveguide filter can handle 11.38 kW and 2.93 kW average power.
5 | PERFORMANCE COMPARISON
The 2.92 mm cables are used for testing, and the calibration is done at the cable end. These cables are connected to VNA
F I G U R E 1 7 Wideband performance of the proposed filter and |
F I G U R E 1 8 Size comparison of the proposed filter and the |
the waveguide filter |
waveguide filter |
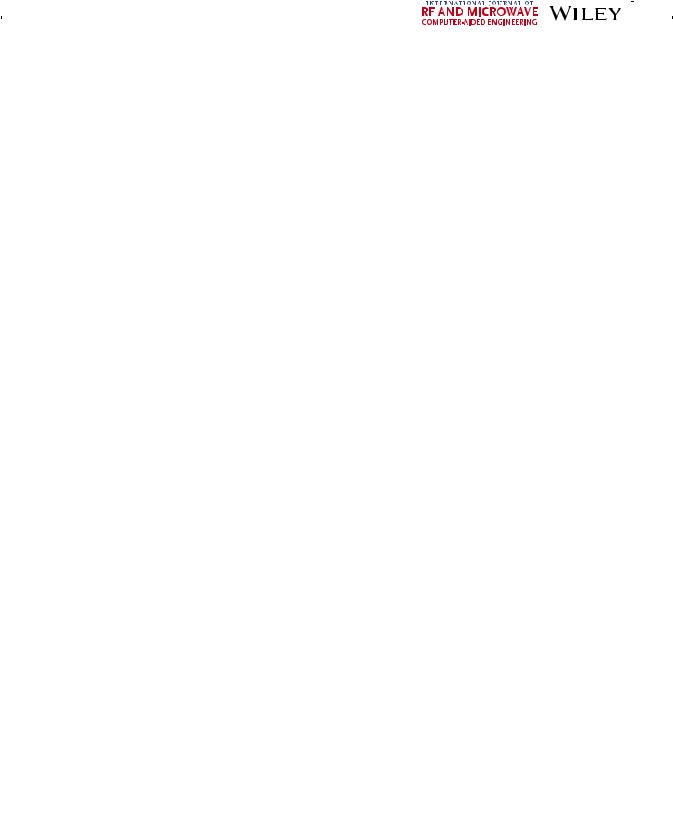
DAD AND GUPTA |
|
|
|
|
9 of 10 |
|
|
|
|
||
|
|
|
|
|
|
|
|
|
|
|
|
|
|
|
|
|
|
at one end and to the adapter at other end. The filters are tested over temperature using WR-28 waveguide to coaxial adapters in the temperature-cycling chamber. The proposed 5-2-0 coaxial resonator filter and the 5-2-0 waveguide filter have tested under the same condition from −20 C to 65 C, and their measured responses have shown in Figure 16. These filters have the stable thermal performance, which meets all specification, but the frequency drift of the proposed filter is less than the waveguide filter. Hence, it offers better design margin than the waveguide filter. The proposed coaxial resonator filter far band rejection is better than waveguide filter as shown in Figure 17. These comparisons tabulated in Table 3 and show in Figure 18.
6 | CONCLUSION
The direct and cross-coupled filters are designed at millimeter-wave band using the higher order high Q TM012 mode of the coaxial resonator. The 5-pole cross-coupled filter has stable RF performance over the temperature. The insertion loss shows that the Q-factor of better than 3000 is achievable practically. The coaxial filters have low loss, and an ultra-wide spurious-free window makes it a potential candidate to be used as a pre-select filter in millimeter wave payload. The different approach of using a non-resonating post for cross-coupling realization in waveguide filter makes the filter compact. The high power analysis is carried out for the identical configuration waveguide and coaxial resonator filter and observed that the peak power handling capacity of the proposed coaxial resonator filter is four times more than the waveguide filter. The coaxial resonator filter offers more design margin than the waveguide filter due to it lower frequency drift. The TM012 mode coaxial resonator provide an alternative to the waveguide resonator due to its better RF and high power handling performance compared to the waveguide resonator.
ACKNOWLEDGMENT
The author acknowledges the support and facility provided by staff of Payload filter division, Mechanical design and fabrication staff at SAC, ISRO for realization of hardware.
ORCID
Vineet K. Dadhttps://orcid.org/0000-0002-6931-7650
REFERENCES
1.LIamas-Garro I, Kim Y, Baek CW, Kim YK. A planar high-Q micromachined monolithic half-coaxial transmission-line filter.
IEEE Trans Microw Theory Tech. 2006;54(12):4161-4168.
2.Wang Y, Ke M, Lancaster MJ. Micromachined 38GHz cavity resonator and filter with rectangular-coaxial feed lines. IET Microw Antenna Propag. 2009;3(1):125-129.
3.Hano K, Kohriyama H, Sawamoto KI. A direct coupled λ/4 coaxial resonator bandpass filter for land mobile communication. IEEE Trans Microw Theory Tech. 1986;34(9):972-976.
4.Morini A, Venanzoni G, Rozzi T. A new adaptive prototype for the design of side coupled coaxial filter with close correspondence to the physical structure. IEEE Trans Microw Theory Tech. 2006; 54(3):1146-1153.
5.Wang X, Jang G, Lee B, Park N. Compact-quad mode bandpass filter using modified coaxial cavity resonator with improved Q- factor. IEEE Trans Microw Theory Tech. 2015;63(3):965-975.
6.Chen FC, Qiu JM, Wong SW, Chu QX. Dual-band coaxial cavity bandpass filter with helical feeding structure and mixed coupling.
IEEE Microw Compon Lett. 2015;25(1):31-33.
7.Yassini B, Yu M. Ka-band dual-mode super Q filters and multiplexers. IEEE Trans Microw Theory Tech. 2015;63(10):33913397.
8.Hung CL, Yeh YS. The propagation constants of higher order modes in coaxial waveguide with finite conductivity. Int J of Infrared and Millimeter Waves. 2005;26(1):29-39.
9.Dad VK, Gupta S. Novel high Q coaxial resonator filter for millimeter wave application. Paper presented at: IEEE MTT-S International Microwave and RF Conference (IMaRC); 2017; Ahmedabad, India
10.Cameron RJ, Mansour RR, Kudsia CM. Microwave Filters for Communication Systems: Fundamental, Design and Applications. Hoboken, NJ, USA: Wiley; 2007.
11.Smith DJ. Filter utilizing a bar coupling. 2001;US Patent 6255919 B1.
12.Cst.com. (2018). CST-computer simulation technology. [online]. https://www.cst.com
13.Ness JB. A unified approach to design, measurement, and tuning of coupled-resonator filters. IEEE Trans Microw Theory Tech. 1998;46(4):343-351.
14.Thomas JB. Cross-coupling in coaxial cavity filters – a tutorial overview. IEEE Trans Microw Theory Tech. 2003;51(4):13681376.
15.Kocbach J, Folgero K. Design procedure for waveguide filters with cross-couplings. Paper presented at: IEEE MTT-S International Microwave Symposium Digest; 2002:1492-1452.
16.Bornemann J, Amari S, Rosenberg U, Vahldieck R. Tolerance analysis of bypass, cross and direct-coupled rectangular waveguide band-pass filters. IEE Proc Microw Antenna Propag. 2005;152(3): 167-170.
17.Amari S, Rosenberg U. Characterstics of cross (bypass) coupling through higher/lower order modes and their applications in elliptic filter design. IEEE Trans Microw Theory Tech. 2005;53(10):31353141.
18.Rosenberg U, Amari S, Bornemann J. Inline TM110 mode filters with high-design flexibility by utilizing bypass couplings of nonresonating TE10/01 modes. IEEE Trans Microw Theory Tech. 2003;51(6):1735-1742.
19.Mohottige N, Oleksandr JU, Budimir D. Ultra compact inline E- plane waveguide Bandpass filter using cross-coupling. IEEE Trans Microw Theory Tech. 2016;64(8):2561-2571.
20.Ruiz-Cruz JA, Zaki KA, Montejo-Garai JR, Rebollar JM. Design of elliptic filters in rectangular waveguide with capacitive and inductive irises and integrated coaxial excitation. Paper presented

10 of 10 |
|
|
|
|
DAD AND GUPTA |
|
|
|
|
||
|
|
|
|
|
|
|
|
|
|
|
|
|
|
|
|
|
|
at: IEEE MTT-S International Microwave Symposium Digest; 2005:1492-1452.
21.Ruiz-Cruz JA, Montejo-Garai JR, Rebollar JM, Zaki KA. Waveguide filters with elliptical function response: overview and results of different implementations. Int J RF and Microw Comp Aid Eng. 2007;17:63-69. https://doi.org/10.1002/mmce.20198.
22.Vaughan JRM. Multipactor. IEEE Trans Electron Devic. 1988;34 (7):1172-1180.
23.Multipaction Design and Test. Noordwijk, The Netherlands: ECSS-E-20-01A Rev.1: European Space Agency; 2013.
24.Woode A, Petit J. Diagnostic investigations into the multipactor effect, Susceptibility zone measurements and parameters affecting a discharge. Eur. Spacy Agency, Noordwijk, The Netherlands: ESTEC Working Paper 1556; 1989.
25.Woo R. Final report on RF voltage breakdown in coaxial transmission lines. Jet Propulsion Lab, CA, U.S.A.:Tech Rep32-1500;1970.
26.Yu M. Introduction to practical aspects of microwave filter design and realization. Proc. IEEE Int,l Microwave Symp.,Workshop WMB:Filter II: Practical Aspects of Microwave Filter Design and Realization. Long Beach; 2005.
27.Yu M. Power-handling capability for RF filters. IEEE Microw Mag. 2007;8(5):88-97.
28.SPARK 3D. [online] Available at: https://www.fest3d.com/rk3d.php
AUTHOR BIOGRAPHIES
Vineet Kumar Dad (SM’2017) was born in Chittorgarh, Rajasthan, India. He Received his Bachelor of Engineering from Engineering College Kota in 2007. He is currently working toward the PhD degree at Dhirubhai Ambani Institute of Information and Communi-
cation Technology, Gandhinagar(Gujarat). He joined Space Applications Centre, ISRO Ahmedabad in 2007 as Scientist/Engineer. He had published four paper in International Conferences. His research interest include dielectric resonator based filter, high Q high performance filter, passive microwave component analysis and design. He is a life member of Astronomical Society of India.
Sanjeev Gupta is currently a Professor and Dean (R&D) at Dhirubhai Ambani Institute of Information and Communication Technology (DA-IICT), Gandhinagar (Gujarat), India. After the graduation of MTech from University of Delhi, he joined SAMEER, IIT
Campus, Powai—an offshoot of TIFR, Mumbai. He was awarded Commonwealth Scholarship by Commonwealth Scholarship Commission, London in 1994. Consequently, he did his PhD at the Queen's University of Belfast, UK in 1997. He has more than 25 years of experience (including 13 years of teaching experience) in the field of High Frequency Microwave and Millimeter Wave Electronics, Antennas, Radars and Communication and Wireless Sub-systems/ Systems from VHF to millimeter wave frequencies. He has authored and published/presented more than 60 publications in reputed National and International Journals and Conferences. He has been the Organizing Committee Member of various conferences held in India and abroad. He is also a reviewer for many reputed national/international journals and conferences. His research interests include Antennas including Active Integrated Antennas and Smart Antennas, Radar and Wireless Communication Sub-systems and Systems, RF/Microwave and Millimeter Wave Applications.
How to cite this article: Dad VK, Gupta S. Design and performance comparison of a novel high Q coaxial resonator filter and compact waveguide filter for millimeter wave payload applications. Int J RF Microw Comput Aided Eng. 2019;e21773. https://doi. org/10.1002/mmce.21773