
диафрагмированные волноводные фильтры / c7f0fa34-e11d-49de-8bcf-6b82e749d239
.pdf3354 |
IEEE TRANSACTIONS ON MICROWAVE THEORY AND TECHNIQUES, VOL. 57, NO. 12, DECEMBER 2009 |
Compact Ridge Waveguide Filters With Arbitrarily
Placed Transmission Zeros Using
Nonresonating Nodes
Mohamed M. Fahmi, Member, IEEE, Jorge A. Ruiz-Cruz,
Raafat R. Mansour, Fellow, IEEE, and Kawthar A. Zaki, Fellow, IEEE
Abstract—Compact size ridge waveguide filters based on the nonresonating node concept are introduced to realize transfer functions with arbitrarily placed attenuation poles. Coupling mechanisms, suitable for the new realization are introduced in diverse -plane and
-plane configurations and with different input-output interfaces. These coupling structures are studied and characterized in detail in order to simplify the design of the proposed filters. Rigorous mode-matching method is used to accelerate the full-wave design of the filter configurations, whose response is also verified using other numerical techniques and experimental data. To validate the proposed novel designs, a prototype has been built and tested. Measurement results agree well with the predicted theoretical simulations.
Index Terms—Attenuation poles, microwave filters, mode matching, ridge waveguides.
I. INTRODUCTION
ECENT advances in modern telecommunication systems R resulted in an ever increasing demand on RF components that satisfy more and more stringent requirements and specifications. Filter with sophisticated transfer functions are being employed with an ever increasing pace. To efficiently utilize the electromagnetic spectrum, filters with very sharp rejection skirts are used. These filters utilize real frequency transmission zeros (TZs) to enhance their rejection characteristics.
The introduction of the extracted pole technique [1] allowed individual control of TZs, but it suffered from its well-known need for phase shifters. The introduction of the nonresonating node (NRN) concept [2] allowed a more modular approach. The NRN concept was utilized in achieving a variety of transfer functions. Numerous designs were realized in rectangular waveguide technology [3], [4] as well as in a variety of other realizations such as combline [5] and coupled lines [6].
Manuscript received August 26, 2009. First published November 03, 2009; current version published December 09, 2009. This work was supported in part by the Ontario Ministry of Research and Innovation and by COM DEV Ltd.
M. M. Fahmi and R. R. Mansour are with the Department of Electrical and Computer Engineering, University of Waterloo, Waterloo, ON, Canada N2L 3G1 (e-mail: mmfahmi@gmail.com; Raafat.Mansour@ece.uwaterloo.ca).
J.A. Ruiz-Cruz is with the Escuela Politécnica Superior, Universidad Autónoma de Madrid, Madrid 28049, Spain (e-mail: Jorge.RuizCruz@uam.es).
K.A. Zaki is with the Department of Electrical and Computer Engineering, University of Maryland at College Park, College Park, MD 20742 USA (e-mail: zaki@umd.edu).
Color versions of one or more of the figures in this paper are available online at http://ieeexplore.ieee.org.
Digital Object Identifier 10.1109/TMTT.2009.2034423
Ridge waveguides present an interesting transmission medium compared to regular rectangular waveguide, owing to the well known fact that the cutoff frequency of their fundamental mode is far lower than that of the rectangular waveguide with same outer dimensions. Another advantage is their wide mono-mode frequency range which allows the realization of very wide band filters with extended spurious free rejection bands [7]–[9].
There has been a noted renewed interest in ridge waveguide filters recently with very interesting and promising results [10], [11]. However the full potential of ridge waveguide technology was not utilized fully due to the fact that the realizations used the ridge waveguides well above their cutoff frequency, at frequencies where the fundamental mode of the outer rectangular enclosure is propagating.
In this paper, we demonstrate novel realizations of very compact ridge waveguide filters with arbitrarily placed TZs by using the NRN concept. In comparison with cross-coupling, the NRN allows independent control of the TZs, which provides more flexibility in achieving the desired transfer function. The basic proposed structure has a NRN with the attached TZ generating cavity [12] in -plane [see Fig. 1(a)]. New coupling configuration in
-plane is also introduced [see Fig. 1(b)]. Both structures may be used to synthesize the NRN in Fig. 1(c). Several designs with four and six poles [see Fig. 1(d)] and different position of the TZs are used to illustrate the proposed structures. The full-wave modeling and design steps of the filters are discussed. Finally, an experimental prototype is used to validate the presented approach.
II.REALIZATION OF NRN IN RIDGE WAVEGUIDE
A. Ridge Waveguide NRN in -Plane Configuration
Fig. 1(a) shows the proposed realization of the NRN and the cavity attached to it, as well as its ideal circuit representation. In this configuration, the NRN and the attached TZ generating cavity are realized by sections of ridge waveguide. They will be arranged in a side-by-side configuration where the coupling mechanism consists of another section of ridge waveguide attaching both the NRN and the attached TZ generating cavity. Other resonators of the filter are also realized by sections of ridge waveguide. The resonators are coupled by sections of evanescent rectangular waveguides which have the same outer dimensions as the ridge waveguide sections. There is a well established procedure for the synthesis of inline filters using this realization [8], [9]. The first proposed design of filters
0018-9480/$26.00 © 2009 IEEE

FAHMI et al.: COMPACT RIDGE WAVEGUIDE FILTERS WITH ARBITRARILY PLACED TZs USING NRNs |
3355 |
Fig. 1. (a) -plane ridge waveguide realization of NRN and the TZ generating cavity. (b) -plane ridge waveguide realization of NRN and the TZ generating cavity. (c) Topology diagram with equivalent circuit parameters. (d) Sample six-pole -plane filter with two TZs.
Fig. 2. Noncanonical waveguide cross sections used to model the structure in Fig. 1(a) (a) Input section. (b) First section of the NRN. (c) Second section of the NRN and the associated resonator. (d) Coupling section between NRN and the associated resonator. From [12].
realizing the NRN and the attached TZ generating cavity in ridge waveguides was reported in [12].
Such realization is amenable to modeling using the well known mode-matching method (MMM) [13], [14], which has three main steps. First, the structure is segmented into a number of uniform waveguides along the longitudinal direction. Second, the generalized scattering matrix of the step between two consecutive waveguides of different cross sections is calculated. In the filters under consideration, besides rectangular waveguides, several ridge waveguides with noncanonical cross sections are obtained, as shown in Fig. 2. Their modes can be calculated following the generalized transverse resonance technique [8]. Finally, all the individual generalized scattering matrices are cascaded to obtain the overall filter response.
B. Ridge Waveguide NRN in -Plane Configuration
In this configuration, the NRN and the attached TZ generating cavity are also realized by sections of ridge waveguide. However, they are arranged in a top-bottom configuration where the coupling mechanism consists of an iris open in the intermediate wall between them. In such case the coupling is achieved by the electric fields which are concentrated between the bottom surface of the ridge and the bottom wall. This configuration provides another alternative for compact ridge waveguide filters. In fact this realization can be useful when used in association with laminated substrates or low-temperature co-fired ceramic (LTCC) technologies [15]. Fig. 3 shows a cut of the proposed realization of the NRN and the cavity attached to it. Its ideal circuit representation is the one shown in Fig. 1(c).
C. Synthesis of NRNs
In the process of designing filters that utilize NRNs, an ideal circuit satisfying the electrical specifications is first obtained [5]. This paper focuses on the physical realization rather than the
Fig. 3. (a) Proposed -plane ridge waveguide realization (cut in half) of NRN and the TZ generating cavity. (b) Side view with dimensions notation. (c) Front view with dimension notation.
ideal circuit synthesis. The scope is to find suitable dimensions of the NRN and the TZ generating cavity such that the structure follows the ideal circuit model of the sub-circuit of the filter network shown in Fig. 1(c). Curve fitting can be used to match the full wave response of the structure to that of the ideal circuit

3356 |
IEEE TRANSACTIONS ON MICROWAVE THEORY AND TECHNIQUES, VOL. 57, NO. 12, DECEMBER 2009 |
Fig. 4. MMM and HFSS response of the structure shown in Fig. 1(a) versus ideal circuit according to Fig. 1(c) with , ,
.
Fig. 6. (a) Proposed ridge waveguide filter using NRN. (b) Topology diagram for the filter. (c) Top view of the proposed filter with dimension notation. Exact dimensions are given in Table I (from [12]).
Fig. 5. Variation of extracted normalized coupling matrix elements with according to Fig. 2(d). All other physical dimensions are fixed with values given in Table I. From [12].
thus identifying the values of the ideal circuit elements corresponding to the physical dimensions. For example, Fig. 4 shows the MMM and HFSS responses of the -plane structure versus the extracted ideal circuit response, where good agreement can be observed.
The extraction procedure was used for the -plane case; Fig. 5 shows how the extracted normalized ideal circuit parameters according to Fig. 1(c) vary with the gap of the ridge section connecting the NRN and the attached resonator keeping all other physical dimensions fixed. Similar procedure can be followed to generate design curves for the
-plane structure.
III. SAMPLE FILTER DESIGNS
Following the design procedure outlined earlier, several different designs are presented. The designs are split into categories based on the location of the TZs with respect to the passband. Filters with TZ below the passband are first introduced, and then filters with TZ above the passband are presented. A filter with zeros on both sides of the passband is also designed for completeness.
The first set of filters assumes ridge waveguide input. This realization is particularly useful in the design of ridge waveguide manifold multiplexers [16]. For experimental verification a design that uses a standard subminiature A (SMA) input tapped in to the input and output ridge waveguide resonators will be presented in Section IV.
A. -Plane Filter With a TZ Below the Passband
The filter has a center frequency of 4 GHz and a 6% fractional bandwidth. It has a maximum in-band return loss of 20 dB and a prescribed zero of transmission located at 3.84 GHz. An ideal circuit satisfying these requirements was obtained according to [5]. The resulting normalized coupling matrix is given in (1) where node 3 is a NRN. The configuration of the filter is given in Fig. 6
(1)
In order to introduce a TZ below the passband, NRNs are realized using sections of ridge waveguides operating between -like and
-like resonances. The whole filter was modeled using MMM. Very good initial dimensions were obtained. This simplified the final full wave optimization of the whole structure. The optimized final response is shown in Fig. 7 versus the ideal circuit response. The dimensions are given in Table I.

FAHMI et al.: COMPACT RIDGE WAVEGUIDE FILTERS WITH ARBITRARILY PLACED TZs USING NRNs |
3357 |
TABLE II
DIMENSIONS (FILTER IN FIG. 8) ACCORDING TO FIGS. 3 AND 8
Fig. 7. Full-wave response of the filter shown in Fig. 6 versus ideal circuit response according to (1) (from [12]).
TABLE I
DIMENSIONS (FILTER IN FIG. 7) ACCORDING TO FIGS. 2 AND 6
Fig. 9. Full-wave response of the filter shown in Fig. 6 versus ideal circuit response according to (1) with signs of the diagonal elements reversed.
Fig. 8. (a) Side view of the proposed filter with dimension notation. (b) Fullwave response of the filter versus ideal circuit response according to (1). Exact dimensions are given in Table II.
B. -Plane Filter With a TZ Above the Passband
The same ideal circuit |
that was previously realized in |
-plane configuration are |
realized in the -plane configu- |
ration shown in Fig. 8(a). The same design methodology is followed and yields a full wave response that matches the sought after ideal circuit response, as shown in Fig. 8(b). The dimensions for this design are given in Table II.
C. -Plane Filter With a TZ Above the Passband
In order to introduce a TZ above the passband, NRNs are realized using sections of ridge waveguides operating between - and
-like resonances.
As an example, a filter with center frequency of 4 GHz and a 6% fractional bandwidth is designed. It has a maximum in-band return loss of 20 dB and a prescribed zero of transmission located at 4.16 GHz. The ideal circuit given in (1) can be used to produce a filter satisfying these requirements with the sole modification of reversing the signs of the diagonal elements in the matrix .
Again, the whole filter is modeled using the MMM. The optimized final response is shown in Fig. 9 versus the ideal circuit response. The dimensions are given in Table III.

3358 |
IEEE TRANSACTIONS ON MICROWAVE THEORY AND TECHNIQUES, VOL. 57, NO. 12, DECEMBER 2009 |
TABLE III
DIMENSIONS (FILTER IN FIG. 9) ACCORDING TO FIGS. 2 AND 6
D. -Plane Filter With TZs on Both Sides of the Passband
In this design a filter with a pair of TZs; one TZ above the passband, and one TZ below the passband will be introduced.
The filter has a center frequency of 5 GHz and its bandwidth extends from 4.9 to 5.1 GHz. The passband has a maximum return loss of 20 dB. The filter has one prescribed zero of transmission located at 4.82 GHz, and another prescribed zero of transmission located at 5.18 GHz.
An ideal circuit satisfying these requirements is obtained according to [5]. The resulting normalized coupling matrix is given in (2), shown at the bottom of this page.
The topology of the proposed filter is shown in Fig. 10. The filter has two NRNs: the first one NRNa (node 2) is operating between - and
-like resonances and the second NRNb (node 6) is operating between
- and
-like resonances.
Again, the whole filter is initially modeled using the MMM. The optimized final response is shown in Fig. 10(c) versus the ideal circuit response. The dimensions are given in Table IV.
IV. EXPERIMENTAL RESULTS
In order to validate the design procedure and simulations, a filter with standard SMA input is designed to facilitate measure-
Fig. 10. (a) Topology diagram for the filter. (b) Top view of the proposed filter with dimension notation. (c) Full-wave response of the filter versus ideal circuit response.
ments. The filter is designed to follow the ideal circuit response given by (1).
In this design, tapping in with an SMA connector was used to achieve the input coupling. MMM modeling of the ridge waveguide tapped-in configuration was reported elsewhere [8]. Fig. 11 shows the layout of the filter with dimensions notations.
The whole structure was optimized with respect to the critical points of the ideal circuit response [17] to reduce the computational cost of the optimization process. The filter was manufactured out of aluminum without any tuning screws. Fig. 12
where
(2)

FAHMI et al.: COMPACT RIDGE WAVEGUIDE FILTERS WITH ARBITRARILY PLACED TZs USING NRNs |
3359 |
TABLE IV
DIMENSIONS (FILTER IN FIG. 10) ACCORDING TO FIGS. 2 AND 10
Fig. 11. (a) Front view of the filter showing the tap-in arrangement. (b) Top view of the filter with dimension convention (from [12]).
shows measured in-band response, in-band insertion loss, and group delay, as well as the wide band response of the filter compared to full-wave response. Good agreement can be observed. The dimensions can be found in [12].
The wide band response shown in Fig. 12(d) shows two spurious peaks surrounding the passband of the filter at approximately 3 and 5.5 GHz. The sources of these two peaks were identified as the -like resonance of the NRN composite section of the filter for the lower frequency peak, and the
-like resonance of the same NRN section. Fig. 13(a) shows the MMM response of the structure shown in Fig. 1(a) over an extended frequency sweep , which shows two peaks correlated with the spurious peaks in the measured response in Fig. 12(d). Fig. 13(b) and (c) shows the resonant field distribution of the NRN at the frequencies of these two peaks.
This spurious problem may be remedied by using alternative discontinuities to realize the NRN behavior. In rectangular waveguide technology, rotated irises [18] and inductive or capacitive septa [19] were used to realize NRNs instead of using cavities operating between and
modes. Similar realizations in ridge waveguide technology may be implemented to overcome the observed spurious resonances.
Fig. 12. (a) Measured in-band response of the filter shown in Fig. 11 versus HFSS response. (b) Measured in-band insertion loss of the filter. (c) Measured group delay of the filter. (d) Measured wide-band response of the filter versus HFSS response. (e) Manufactured filter without cover.

3360 |
IEEE TRANSACTIONS ON MICROWAVE THEORY AND TECHNIQUES, VOL. 57, NO. 12, DECEMBER 2009 |
Fig. 13. (a) MMM response of the structure shown in Fig. 1(a). (b) Resonant electric field distribution along the structure shown in Fig. 1(a) at 3 GHz.
(c) Resonant electric field distribution along the structure shown in Figs. 1(a) at5.5 GHz.
V. CONCLUSION
Novel realizations of ridge waveguide filters based on the NRN concept are presented in both -plane and
-plane configurations. In the
-plane configuration, a simplified coupling mechanism utilizing all ridge waveguide structure is used. In the
-plane structure, coupling through an iris in the intermediate wall is used. Different filter responses with prescribed TZs on both sides of the passband are designed, allowing transfer functions with high selectivity while maintaining the compact size provided by the ridge waveguides. A prototype with tapped-in coaxial excitation was built and measured to verify the design approach. Good agreement between the measured response and full-wave model response can be observed.
ACKNOWLEDGMENT
The authors would like to acknowledge Dr. Y. Zhang, Radio Frequency Systems (RFS), for help with ideal circuit synthesis.
REFERENCES
[1]J. D. Rhodes and R. J. Cameron, “General extracted pole synthesis
technique with applications to low loss mode filters,” IEEE Trans. Microw. Theory Tech., vol. MTT-28, no. 9, pp. 1018–1028, Sep. 1980.
[2]S. Amari and U. Rosenberg, “Synthesis and design of novel inline filters with one or two real transmission zeros,” IEEE Trans. Microw. Theory Tech., vol. 52, no. 5, pp. 1464–1478, May 2004.
[3]S. Amari, U. Rosenberg, and J. Bornemann, “Singlets, cascaded singlets and the nonresonating node model for advanced modular design of elliptic filters,” IEEE Microw. Wireless Compon. Lett., vol. 14, no. 5, pp. 237–239, May 2004.
[4]S. Cogollos, R. J. Cameron, R. R. Mansour, M. Yu, and V. E. Boria, “Synthesis and design procedure for high performance waveguide filters based on nonresonating nodes,” in IEEE MTT-S Int. Microw. Symp. Dig., Jun. 2007, pp. 1297–1300.
[5]S. Amari and G. Macchiarella, “Synthesis of inline filters with arbitrarily placed attenuation poles by using nonresonating nodes,” IEEE Trans. Microw. Theory Tech., vol. 53, no. 10, pp. 3075–3081, Oct. 2005.
[6]S. Cogollos, V. E. Boria, R. J. Cameron, and R. R. Mansour, “Design procedure for low cost substrate microstrip filters based on nonresonating nodes,” in IEEE MTT-S Int. Microw. Symp. Dig., Jun. 2008, pp. 543–546.
[7]Y. Rong, K. A. Zaki, J. Gipprich, M. Hageman, and D. Stevens, “LTCC wide-band ridge-waveguide bandpass filters,” IEEE Trans. Microw. Theory Tech., vol. 47, no. 9, pp. 1836–1840, Sep. 1999.
[8]J. A. Ruiz-Cruz, M. E. Sabbagh, K. A. Zaki, J. Rebollar, and Y. Zhang, “Canonical ridge waveguide filters in LTCC or metallic resonators,”
IEEE Trans. Microw. Theory Tech., vol. 53, no. 1, pp. 174–182, Jan. 2005.
[9]J. A. Ruiz-Cruz, Y. Zhang, K. A. Zaki, A. J. Piloto, and J. Tallo, “Ultra wideband LTCC ridge waveguide filters,” IEEE Microw. Wireless Compon. Lett., vol. 17, no. 2, pp. 115–117, Feb. 2007.
[10]S. Bastioli, L. Marcaccioli, and R. Sorrentino, “Novel waveguide pseudo-elliptic fiters using slant ridge resonators,” in IEEE MTT-S Int. Microw. Symp. Dig., Jun. 2007, pp. 619–622.
[11]S. Bastioli, L. Marcaccioli, and R. Sorrentino, “A novel class of compact dual-mode rectangular waveguide filters using square ridge resonators,” in Proc. 38th Eur. Microw. Conf., Oct. 2008, pp. 626–629.
[12]M. Fahmi, J. A. Ruiz-Cruz, R. R. Mansour, and A. Zaki, “Compact ridge waveguide filters using non-resonating nodes,” in IEEE MTT-S Int. Microw. Symp. Dig., Jun. 2009, pp. 1337–1340.
[13], T. Itoh, Ed., Numerical Techniques for Microwave and MillimeterWave Passive-Structures. New York: Wiley, 1989.
[14]G. Conciauro, M. Guglielmi, and R. Sorrentino, Advanced Modal Analysis: CAD Techniques for Waveguide Components and Filters. New York: Wiley, 1999.
[15]M. Fahmi, J. A. Ruiz-Cruz, K. A. Zaki, and A. J. Piloto, “LTCC wideband canonical ridge waveguide filters,” in IEEE MTT-S Int. Microw. Symp. Dig., Jun. 2005, pp. 249–252.
[16]Y. Zhang, J. A. Ruiz-Cruz, and K. A. Zaki, “Ridge waveguide divider junctions for wide-band multiplexer applications,” in IEEE MTT-S Int. Microw. Symp. Dig, Jun. 2006, pp. 1225–1228.
[17]R. J. Cameron, R. R. Mansour, and C. Kudsia, Microwave Filters for Communication Systems: Fundamentals, Design and Applications. New York: Wiley, 2007.
[18]S. Amari and U. Rosenberg, “New in-line dual and triple-mode cavity filters with non-resonating nodes,” IEEE Trans. Microw. Theory Tech., vol. 53, no. 4, pp. 1272–1279, Apr. 2005.
[19]Macchiarella and G. Politi, “Use of generalized coupling coefficients in the design of extracted-poles waveguide filters with non-resonating nodes,” in IEEE MTT-S Int. Microw. Symp. Dig., Jun. 2009, pp. 1341–1344.
Mohamed M. Fahmi (S’05–M’09) received the B.Sc. degree (with honors) in electronics engineering from Mansoura University, Mansoura City, Egypt, in 1999, the M.S. degree from Howard University, Washington, DC, in 2003, and the Ph.D. degree from the University of Maryland at College Park, in 2007.
From 1999 to 2000, he was a Teaching/Research Assistant with the Department of Electronics Engineering, Mansoura University. From 2001 to 2003, he was a Research Assistant with the Department of Electrical and Computer Engineering, Howard Uni-
versity. From 2003 to 2007, he was a Teaching/Research Assistant with the Department of Electrical and Computer Engineering, University of Maryland at College Park. From January to July 2008, he was a Post-Doctoral Researcher with the Department of Electrical and Computer Engineering, University of Maryland at College Park. In September 2008, he joined the Department of Electrical and Computer Engineering, University of Waterloo, Waterloo, ON, Canada, where he is currently a Post-Doctoral Fellow. His current research interests include CAD of microwave devices.
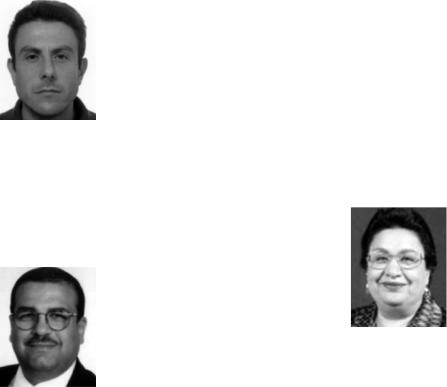
FAHMI et al.: COMPACT RIDGE WAVEGUIDE FILTERS WITH ARBITRARILY PLACED TZs USING NRNs |
3361 |
Jorge A. Ruiz-Cruz received the Ingeniero de Telecomunicación degree and Ph.D. degree from the Universidad Politécnica de Madrid (UPM), Spain, in 1999 and 2005, respectively.
Since 1999, he has collaborated with the Grupo de Electromagnetismo Aplicado y Microondas, UPM. Prior to beginning the doctoral program, he was with RYMSA (2000). He has visited the Politecnico di Milano, Milan, Italy (2002), the University of Maryland at College Park (several stays from 2003 to 2008), the Ecole Polytechnique Fédérale de
Lausanne, Lausanne, Switzerland (2007), and the University of Waterloo, Waterloo, ON, Canada (2009). Since 2006, he has been with the Universidad Autónoma de Madrid, Madrid, Spain, where in 2009 he became an Associate Professor. His current research interests are CAD of microwave passive devices and circuits (filters, multiplexers, ortho-modes, etc.).
Dr. Ruiz-Cruz was the recipient of an award from the Telefónica FoundationCOIT (Spanish college for telecommunication engineers) for his doctoral thesis. He also received an award from the UPM.
Raafat R. Mansour (S’84–M’86–SM’90–F’01) was born in Cairo, Egypt, on March 31, 1955. He received the B.Sc (with honors) and M.Sc degrees from Ain Shams University, Cairo, Egypt, in 1977 and 1981, respectively, and the Ph.D. degree from the University of Waterloo, Waterloo, ON, Canada, in 1986, all in electrical engineering.
In 1981, he was a Research Fellow with the Laboratoire d’Electromagnetisme, Institut National Polytechnique, Grenoble, France. From 1983 to 1986 he was a Research and Teaching Assistant
with the Department of Electrical Engineering, University of Waterloo. In 1986, he joined COM DEV Ltd., Cambridge, ON, Canada, where he held several technical and management positions with the Corporate Research
and Development Department. In 1998, he became a Scientist. In January 2000, he joined the University of Waterloo, as a Professor with the Department of Electrical and Computer Engineering. He holds a Natural Sciences and Engineering Research Council of Canada (NSERC) Industrial Research Chair in RF engineering with the University of Waterloo. He is the Founding Director of the Center for Integrated RF Engineering (CIRFE), University of Waterloo. He has authored or coauthored numerous publications in the areas of filters and multiplexers, high-temperature superconductivity and microelectromechanical systems (MEMS). He coauthored Microwave Filters for Communication Systems (Wiley, 2007). He holds several patents related to areas of dielectric resonator filters, superconductivity, and MEMS devices. His current research interests include MEMS technology and miniature tunable RF filters for wireless and satellite applications.
Dr. Mansour is a Fellow of the Engineering Institute of Canada (EIC).
Kawthar A. Zaki (SM’85–F’91) received the B.S. degree (with honors) from Ain Shams University, Cairo, Egypt, in 1962, and the M.S. and Ph.D. degrees from the University of California at Berkeley, in 1966 and 1969, respectively, all in electrical engineering.
From 1962 to 1964, she was a Lecturer with the Department of Electrical Engineering, Ain Shams University. From 1965 to 1969, she was a Research Assistant with the Electronics Research Laboratory, University of California at Berkeley. In 1970, she
joined the Electrical Engineering Department, University of Maryland at College Park, where she is currently a Professor Emeritus of electrical engineering. She has authored or coauthored over 200 publications. She holds five patents on filters and dielectric resonators. Her research interests are in the areas of electromagnetics, microwave circuits, simulation, optimization, and CAD of advanced microwave and millimeter-wave systems and devices.
Prof. Zaki was the recipient of several academic honors and awards for teaching, research, and inventions.