
диафрагмированные волноводные фильтры / 3a102f49-771e-4453-b60d-41b6bb6fb31e
.pdfNew Architectures for Planar Filters Using Split-Ring Resonators
A. León
Facultad Ingeniería Eléctrica, Cujae.
La Habana, Cuba alainleon@electrica.cujae.edu.cu
Abstract—— An improved technique is outlined to design filters in planar configurations. The new procedure is applied to enhance the performance of some filters in planar configuration. This paper illustrates that complementary split-ring resonators (CSRRs), electrically coupled to a microstrip transmission line and split-ring resonators (SRRs) magnetically coupled to a finline structure can be applied to improve the design of such filters. For this propose, classical variants of millimeter wave filters have been analyzed. These components include microstrip low-pass filters, finline bandpass/bandstop filters and antipodal finline bandstop filters. The properties of these configurations are discussed; the experimental data are in good agreement with the simulations. The results show the usefulness and validity of the new structures as it is possible to achieve a very low insertion loss and very sharp transition bands with high rejection capabilities.
Keywords-component: Planar filters, Split-ring resonators
I.INTRODUCTION
Nowadays, circuits that operate in microwave and millimeter wave bands are required to be greatly miniaturized while having and to have a good performance. One of the most up-to-date fields of research is the filtering of certain frequency bands for suppressing spurious signal and undesired harmonics in microwave circuits [1-4]. Split-ring resonators (SRR) are compact structures with easily scalable parameters, which provide a powerful alternative to EBGs (Electromagnetic band gap structures) that have been under study for several years [5- 9]. Split ring resonators (SRRs) together with complementary split ring resonators (CSRRs) contribute to maintaining the smaller size of the passband filter performance as the sub wavelength dimension enables sharp rejection [10-18]. Although a variety of filter configurations has been developed to reduce the level of harmonic signals, in this work the authors design a step-impedance low-pass filter (SILPF) using asymmetrical stepped microstrip structures. However, in addition, the incorporation of CSRRs is proposed. By incorporating CSRRs, it is demonstrated that it is feasible to produce a filter that performs with no ripples in the passband, while producing a sharp stop band rejection in the vicinity of its resonant frequency. This paper expands the above procedure to finline filter design. SRRs are sub wavelength resonators that are able to inhibit signal propagation in a narrow band in the vicinity of their resonant frequency, due to the magnetic field is polarized along the ring's axis and the finline structures present an appropriate magnetic field distribution for this purpose. Finline technology has been established as a practical approach in the design and construction of mm-wave components and subsystems. During recent years, various
A. Casanueva, A. Mediavilla, and J. Herrero
DICOM, University of Cantabria Santander, Spain
alicia.casanueva@unican.es
finline filters have been widely analyzed [19-20]. However they cannot provide wide stop band with high attenuation level. Since higher order modes occur between the discontinuities, reducing the discontinuities is crucial in the function of the filter. To this end, this paper aims to find alternatives to conventional filters mainly on finline slot sharp discontinuities. Considering that among the various finline structures, the antipodal line structure provides very large bandwidth [21], a band stop filter (BSF) with extremely wide stop band has been developed. This work has been organized as follows. Section II optimizes the SILPF in microstrip technology using CSRRs. Once the two objectives of reducing the circuit size and significantly pushing the spurious to higher frequency had been achieved, the next step was to improve the above results. To this end, a novel model of SILPF and its complementary structure (CSRRs) are presented and analyzed. The measured results of the filter prototype are in good agreement with the simulated results [22] and the responses of the prototype filters show a wide stopband with high attenuation, a high selectivity, and a small ripple level in the pass band, which is attributed to the etched resonators in the ground plane. Section III is devoted to the use of SRRs in the filter design of BPF/BSF finline structures. A band pass filter with a finline structure and a band stop filter in antipodal configuration are proposed. The analysis process has been very similar to that in microstrip technology. After the results were obtained, it was thought that these SRRbased structures could be of interest for the design of planar filters. The results obtained using a resonant ring set suggests that these elements and their duals could provide tools that would dramatically improve the response of filters in planar technology. The theoretical and experimental results were obtained using the full–wave EM simulator Ansoft HFSS and HP8510 network analyzer.
II.MICROSTRIP FILTERS USING CSRRS
A.Microstrip configuration
The purpose of this section is to extend the analysis to design a microstrip low-pass filter using complementary ring resonators oriented at an arbitrary angle θ [22]. The main goal here is to see how the performance of a low-pass filter improves in microstrip technology, incorporating complementary ring resonators. Figure 1 depicts the topology of a microstrip low-pass filter.
978-1-4244-7057-0/10/$26.00 ©2010 IEEE
394

Figure 1. Schematic view of the proposed microstrip- low-pass filter.
By etching the CSRR underneath the ground plane below the SILPF it can be ensured that the particle is excited properly. This is achieved by an electric field polarized in the axial direction of the ring. Around the resonant frequency a deep stopband is introduced due to the presence of an effective negative permittivity. A CSRR is the negative image of a split ring resonator (SRR) and it produces a sharp rejection stop band in the vicinity of its resonant frequency. The correct behavior of the CSRR cell basically depends on three factors: the geometry, the dielectric and the position of the CSRR in the ground of the SILPF. First we designed a CSRR. The dimensions were chosen in such a way that CSRR’s first resonant frequency is equal to the -3 dB cutoff frequency of the SILPF which is required to be at 3 GHz. The design and calculation of the resonant frequency of the CSRR is not discussed here since a detailed explanation is given in [13]. Figure 2 shows a typical layout and Table 1 shows the geometric parameters. Once the classical structure of the filter has been validated, see Figure 5, the next step is to appropriately position a CSRR suitable for improving the response of the filter above.
Figure 2. Geometry of the unit cell of CSRR.
Table 1 Dimensions of the double CSRR
Parameters |
Dimensions (mm) |
r1 |
0.41051 |
r2 |
1.21051 |
r3 |
2.6 |
r4 |
2.6 |
c |
0.4 |
d |
0.6 |
g |
0.4 |
Substrate |
εr = 3.2, (δ = 0.003) |
(L x W x H) |
22.19 x 20.926 x 0.51 |
Figure 3. 50 ohms microstrip line loaded with CSRR.
Figure 4. Electromagnetic field distribution for the first two resonant frequencies, 3.04 GHz and 9.89 GHz. (a) Electric field, (b) Magnetic field.
Once it had been seen how the resonant frequency of the CSRR could be tuned with the help of device dimensions, the second step was to place this structure in the correct position in the ground plane of the filter. This filter has special properties: is possible to change the scattering parameters modifying the radius of the resonators both in the top wall and on the bottom, R and r respectively. This flexibility is a very important property if the optimization of the filter is desired Figure 5 shows the top filter layout and Table 2 the geometric dimensions.
Figure 5. Top layout
Table 2 Dimensions of the SILPF
Parameters |
Dimensions mm) |
r1 |
2.9 |
r2 |
1.205 |
l1 |
5.0 |
l2 |
3.19 |
l3 |
2.276 |
w1 |
1.2266 |
w2 |
0.14 |
w3 |
1.034 |
395

Figure 6. Magnitude of the scattering parameters versus frequency for two different orientations of CSRR.
Figure 6 illustrates that this filter produces a sharp rejection stop band in the vicinity of its resonant frequency and the return loss depends on the CSRR orientation, achieving a very flat response in the stop band when the resonators are rotated by an angle θ=90 degrees.
In Figure 7 it is possible to observe how the electric field orientation and concentration is modified according to the frequency operation: a) before resonant frequency 2.93 GHz, b) at the CSRR resonant frequency 3.08 GHz and c) after resonant frequency 3.23 GHz.
(a) |
(b) |
Figure 8. Finline structures: a) Unilateral finline, b) Antipodal finline.
The same design procedure applied in microstip LPF using CSRRs can be applied to design a finline Bandpass/Stopband filter in finline configuration using SRRs. In this paper, it will be illustrated that split-ring resonator (SRRs) pairs can be used as elements to improve the response of filters in finline configurations. For this purpose, firstly, the conventional band pass filter method is used. Once the simulated electromagnetic response is found, a cell of SRR is placed in the finline filter structure as shown in Figure 9.
(1) |
(2) |
Figure 7. Electric field distribution. 1) Transversal section, 2) Frontal view on the substrate’s bottom face.
III.FINLINE FILTERS USING SRRS
A.Finline configuration.
The novel technique for developing a finline BPF and antipodal BSF is presented in this paper. Figure 8a and Figure 8b show the unilateral and antipodal configurations respectively. This section discusses various filters in unilateral finline and antipodal finline. Here due to the magnetic field distributions in the finline structures, the SRRs are excited in a more effective way.
(a) |
(b) |
(c)
Figure 9. a) Transmission loss and layout of the: E-plane finline filter in WR90 loaded with SRRs, b) Return loss, c) Transmission loss for different locations of the SRRs.
The proposed structure demonstrates a wide stop band with high attenuation, a high selectivity, and a small ripple level in the pass band, which is attributed to the double ring wire resonators. After that, the resonator was placed on the lower face of the substrate and the response was similar Figure 9c. Tables 3 and 4 present the geometric and electrical parameters.
The proposed structure provides a wide stop band with high attenuation, high selectivity and a small ripple level in the pass band, which is attributed to the double ring wire resonators.
396

Table 3 Dimensions of the split ring resonator (SRR)
Parameters |
Dimensions (mm) |
r |
2.425 |
c |
0.533 |
d |
0.435 |
g |
0.4 |
Table 4 Dimensions of the finline Band Pass Filter |
|
|
|
Parameters |
Dimensions (mm) |
Width of the waveguide, a |
22.86 |
Width of substrate, w1 |
10.16 |
Width of slot, w2 |
8.16 |
Total length of the |
42.058 |
waveguide filter, L4 |
|
metallization thickness, t |
0.035 |
Length of resonator unit, |
|
L1 |
11.466 |
L2 |
12.216 |
L3 |
11.466 |
Length of metallic septum, S1 |
0.493 |
S2 |
2.962 |
S3 |
2.962 |
S4 |
0.493 |
Substrate |
εr = 3.2, (δ = 0.003 |
(L x W x H) |
42.058 x 8.16 x 1.56 |
The next step in this work involved the design of two new prototypes of BSF using SRRs in finline and antipodal configurations. The first one was designed using 5 SRR cells. Figure 10a illustrates the transmission parameter as a function of frequency together with the geometry of the filter and Figures 10b and 10c depict the electrical and magnetic field at two different frequencies near stop band and pass band, respectively.
The dimensions of the above filter are shown in Tables 5-6
Table 5 Dimensions of the split ring resonator (SRR)
Parameters |
Dimensions (mm) |
|
r |
2.085 |
|
c |
0.4 |
|
d |
0.3 |
|
g |
0.3 |
|
Table 6 Dimensions of the finline Band Stop Filter |
||
|
|
|
Parameters |
Dimensions (mm) |
|
Width of the waveguide, a |
22.86 |
|
Width of substrate W1 |
14.2 |
|
Total length LT |
60 |
|
Length of the filter, L1, |
53.408 |
|
With metal strip, w |
1.135 |
|
Metallization thickness, t |
0.002 |
|
Separation between resonators , |
1.00 |
|
d |
|
|
Substrate |
60 x 10.16 x 0.51 |
|
(L x W x H) |
Neltec NX9245 |
εr = 2.45 |
The second BSF prototype was built using 4 SRR cells in each substrate face of the finline waveguide. Figure 11 shows this filter.
The function used to describe the finline taper follows a square sine distribution.
W(z) = [(b + w) 2] × sin2 (πz 2L) |
(1) |
where b, w and L are constants numerically adjusted in order to achieve the desired taper height.
|
c |
εr |
b |
h
a
a = 22.86 mm b = 10.86 mm C = 2.02 mm h = 1.56 mm
εr = 3.2
Figure 10a. Insertion loss of a BSF in finline and its layout.
(a)
(b)
Figure 10b. (a) Electric (b) magnetic field distribution in the stop band (8.7GHz)
(a)
(b)
Figure 10c. Electric (a) and magnetic (b) field distribution in the pass band (12GHz).
Figure 11. BSF in finline structure.
Tables 7 and 8 present the geometric and electrical parameters.
Table 7 Dimensions of the split ring resonator (SRR)
Parameters |
Dimensions (mm) |
r |
1.55 |
c |
0.3 |
d |
0.3 |
g |
0.3 |
397

Table 8 Dimensions of the finline Band Stop Filter
Parameters |
Dimensions (mm) |
Width of the waveguide, a |
19 |
Height of the W (with groove), h |
11.2515 |
Height of the W (without groove) |
9.5 |
|
|
Total length LT |
120 |
Length of the filter, LF, |
82.097 |
Width of the filter, h |
11.2512 |
Width of metal strip and groove, w1 |
0.8756 |
Width metal strip, w2 |
2.5502 |
Metallization thickness, t |
0.0017 |
Separation between resonators |
0.6 |
Substrate |
82.097 x 11.2512 x 0.254 |
(L x W x H) |
Arlon CuClad 217 εr = 2.17 |
Figure 12 presents the excellent results obtained and the good agreement between measured and simulated results in both phase and scattering parameters.
(a)
(b)
(c)
Figure 12. Measured and simulated scattering parameters versus frequency. (a) Magnitude of the return loss; (b) Magnitude of the insertion loss;
(c) Phase of the insertion loss versus frequency.
Given the good results obtained previously, we wanted to extend the previous configuration to the design of a filter in antipodal configuration in order to see how varying the dimensions of the resonators may shift the band of the filter, since the antipodal configuration presents greater bandwidth and more design flexibility. This section describes an E-plane antipodal finline band stop filter, in which SRR dimensions have been adjusted. The results are shown in Figures 13. Figure 13a presents the scattering parameters and, Figure 13b shows the variation of the filter response by changing the SRR radius to illustrate the flexibility of these types of structures. Finally, Figure 13c depicts the electromagnetic field and density current distributions. Table 9 presents the dimensions of the resonators. Table 10 gives the geometric parameters values of the antipodal finline.
Table 9 Dimensions of the split ring resonator (SRR)
Parameters |
Dimensions (mm) |
r |
2.085 |
c |
0.4 |
d |
0.3 |
g |
0.3 |
Table 10 Dimensions of the antipodal Band Stop Filter
Parameters |
Dimensions (mm) |
|
Width of the waveguide, a |
22.86 |
|
Width of substrate W1 |
8.16 |
|
Total length LT |
60 |
|
Length of the filter, L1, |
53.408 |
|
With metal strip, w |
1.135 |
|
Metallization thickness, t |
0.002 |
|
Separation between resonators , d |
1.00 |
|
Substrate |
60 x 10.16 x 0.51 |
|
(L x W x H) |
Neltec NX9245 |
εr = 2.45 |
(a)
(b)
398
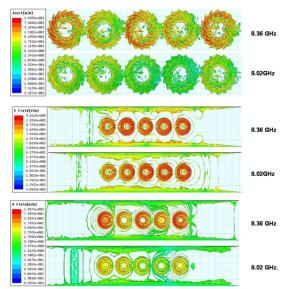
(c)
Figure 13. S-parameter magnitude of (a) the antipodal finline BSF (b) two different antipodal BSF structures. (c) Density current distribution as a function of frequency.
IV. CONCLUSIONS
By incorporating CSRRs, we demonstrate that it is feasible to produce a filter that performs with no ripples in the passband, while producing a sharp stop band rejection in the vicinity of its resonant frequency. In summary, after comparing both the numerical and experimental data, we can conclude that this SILPF combined with CSRRs is compact in size and can provide a deep and ultra-wide stopband, high selectivity and low ripple level in the passband. Moreover, it was demonstrated that the presence of SRRs in finline structures, due to their excellent performance, can be easily applied to circuit applications requiring filtering functionality.
ACKNOWLEDGMENT
The authors would like to thank the Spanish Ministry of Science and Innovation (MICINN) for the financial support provided through project TEC2008-06684-C03-01 and CONSOLIDER-INGENIO 2010 CSD2008-00068.
REFERENCES
[1]J.-W. Sheen, “A compact semi-lumped low-pass filter for harmonics and spurious suppression,” IEEE Microw. Wireless Compon Lett., vol. 10, no. 3, pp. 92–93, Mar. 2000.
[2]Lopetegi, T., M. A. G. Laso, J. Hernandez, M. Bacaicoa, D. Benito, M. J. Garde, M. Sorolla, and M. Guglielmi, “New microstrip “wiggly-line” filters with spurious passband suppression,” IEEE Trans. Microw. Theory Tech., Vol. 49, No. 9, 1593–1598, 2001.
[3]Kuo, J.-T., W.-H. Hsu, and W.-T. Huang, “Parallel coupled microstrip filters with suppression of harmonic response,” IEEE Microwave Wireless Compon. Lett., Vol. 12, No. 10, 383–385, 2002.
[4]Kim, B. S., J. W. Lee, and M. S. Song, “An implementation of harmonic-suppression microstrip filters with periodic grooves,” IEEE Microwave Wireless Compon. Lett., Vol. 14, No. 9, 413–415, 2004.
[5]Yang, F. R., K. P. Ma, Y. Qian, and T. Itoh, “A uniplanar compact photonic-bandgap (UC-PBG) structure and its applications for microwave circuits,” IEEE Trans. Microwave Theory Tech., Vol. 47, No. 8, 1509–1514, 1999.
[6]Ahn, D., Park, J.-S., Kim, C.-S., Qian, Y., and Itoh, T., “A design of lowpass filter using the novel microstrip defected ground structure”, IEEE Trans. Microw. Theory Tech., 2001, 49, (1), pp. 86–93.
[7]Qi, L., H. M. Salgado, A. M. Moura, and J. R. Pereira,”Dualband antenna design using an EBG artificial magnetic conductor
ground plane”, IEEE Transactions on Antennas and Propagation Conference, 2008. LAPC 2008. Loughborough, 217-220, 2008.
[8]Zheng, Q.-R., B.-Q. Lin, Y.-Q. Fu, and N.-C. Yuan, “Characteristics and applications of a novel compact spiral electromagnetic band-gap (EBG) structure,” Journal of Electromagnetic Waves and Applications, Vol. 21, No. 2, 199-213, 2007.
[9]Santanu Dwari and Subrata Sanyal, “Compact wide stopband low-pass filter using rectangular patch compact microstrip resonant cell and defected ground structure”, Microwave and Optical Technology Letters (MOTL), Interscience Wiley, Vol. 49, No. 4, pp. 798-800, April 2007.
[10]Pendry, J. B., A. J. Holden, D. J. Robbins, et al.,”Magnetism from conductors and enhanced nonlinear phenomena”, IEEE Trans. Microwave Theory Tech., Vol. 47, No. 11, 2075, 2084, 1999.
[11]Gay-Balmaz, P. and O. J. F. Martin, “Electromagnetic resonances in individual and coupled split-ring resonators,” Journal of Applied Physics, Vol. 92, No. 5, 2002, 2929-2936.
[12]R. Marqués, F. Mesa, J. Martel and F. Medina, “Comparative analysis of edge and broadside couple split ring resonators for metamaterial design. Theory and Experiment”, IEEE Trans. Ant. Propag., vol. 51, 2003 pp.2572-2581.
[13]J.D. Baena, J. Bonache, F. Martín, R. Marqués, F. Falcone, T. Lopetegi, M.A.G. Laso, J. García, I Gil and M. Sorolla, "Equivalent circuit models for split ring resonators and complementary split rings resonators coupled to planar transmission lines", IEEE Transactions on Microwave Theory and Techniques, vol. 53, 2005, pp. 1451-1461.
[14]Bonache, J., Marin, F., Falcone, F., Garcia, J., Gil, I., Lopetegi, T., Laso, M.A.G., Marques, R., Medina, F., and Sorolla, M.,”Super compact split ring resonators CPW band pass filters”. IEEE MTT-S Int. Microwave Symp. Dig., Fort Worth, TX, USA, June 2004.
[15]Bonache, J., F. Martin, F. Falcone, et al., “Application of complementary split-ring resonators to the design of compact narrow band-pass structures in microstrip technology”, Microwave and Optical Technology Letters, Vol. 46, No. 5, 508-512, 2005.
[16]Garcia-Garcia, J., Martin, F., Falcone, F., Bonache, J., Baena, J.D., Gill, I.,Amat, E., Lopetegi, T., Laso, M.A.G., Iturmendi, J.A.M., Sorolla, M., and Marques, R., “Microwave filters with improved stopband based on sub-wavelength resonators”, Microw. Opt. Tech. Lett., 2005, 46, (3),pp. 283–286.
[17]Bonache, J., Martin, F., Garcia-Garcia, J., Gil, I., Marques, R., and Sorolla, M., “Ultra wide band pass filters (UWBPF) based on complementary split ring resonators”, IEEE Antennas Wirel. Propag. Lett., 2005, 1A.
[18]Baena, J.D., Bonache, J., Martin, F., Marques, R., Falcone, F.,Lopetegi, T., Laso, M.A.G., Garcia, J., Gill, I., and Sorolla, M.,”Equivalent-circuit models for split ring resonators coupled to planar transmission lines”, IEEE Trans. Microw. Theory Tech., 2005, 53, (4), pp. 1451–1461.
[19]F. Arndt, J. Bornemann, D. Grauerholz, and R. Vahldieck, “Theory and Design of Low Insertion Loss Fin-Line Filters,” IEEE Trans. Microwave Theory Tech., Vol. MTT-30, Feb. 1982, pp. 155-163.
[20]Bharathi Bhat and Shiban K. Koul, “Analysis Design and Applications of Fin Lines”, Artech House, 1987.
[21]A. M. K. Saad, J. D. Miller, A. Mitha and R. Brown, “Analysis of Antipodal Ridge Waveguide Structure and Application on Extremely Wide Stopband Lowpass Filter”, IEEE MTT-S Int. Micr. Symp. Digest, Baltimore, MD, June 1986, pp. 361-363.
[22]A. Casanueva, A. León, O. González, A. Mediavilla, M. Arias, N. Amar, “Improved Compact Microstrip Low Pass Filter With Novel Distributions Of Complementary Split Ring Resonators (CSRRs)”, AsiaPacific Microwave Conference (APMC 2009). December 7-10, 2009, Singapore. pp. 1450-1453.
399