
диафрагмированные волноводные фильтры / fcf43c6c-5f80-497c-bffb-577033acff1c
.pdf
See discussions, stats, and author profiles for this publication at: https://www.researchgate.net/publication/268357621
LIQUID CRYSTAL BASED RECONFIGURABLE ANTENNA ARRAYS
Article
CITATIONS |
|
READS |
9 |
|
2,343 |
7 authors, including: |
|
|
Onur Hamza Karabey |
Rolf Jakoby |
|
Technische Universität Darmstadt |
Technische Universität Darmstadt |
|
55 PUBLICATIONS |
801 CITATIONS |
569 PUBLICATIONS 7,646 CITATIONS |
SEE PROFILE |
|
SEE PROFILE |
Atsutaka Manabe |
|
|
53 PUBLICATIONS |
712 CITATIONS |
|
SEE PROFILE |
|
|
All content following this page was uploaded by Atsutaka Manabe on 21 October 2015.
The user has requested enhancement of the downloaded file.

LIQUID CRYSTAL BASED RECONFIGURABLE ANTENNA ARRAYS
Onur Hamza Karabey1, Saygin Bildik1, Carsten Fritzsch1, Sebastian Strunck1, Alexander Gaebler1,
Rolf Jakoby1, and Atsutaka Manabe2
1Microwave Engineering Group, Technische Universitaet Darmstadt, Merckstr. 25, 64283, Darmstadt, Germany,
2Merck KGaA, Liquid Crystal Division, Physical Department , Frankfurter Strasse 250, 64293, Darmstadt, Germany , Email : karabey@mwt.tu-darmstadt.de
ABSTRACT
This paper presents reconfigurable antenna arrays based on liquid crystals (LCs) for earth and space applications. Tunable reflectarrays at 35 GHz and tunable phased arrays for Kuand Ka-bands are discussed in detail in terms of technology, performance, size and cost. Particular attention is given to LC based cost effective planar phase shifters in order to design multilayer reconfigurable phased array antennas applicable for, i.e., automotive applications.
Key words: Microstrip antennas, liquid crystal devices, phase shifters, reconfigurable architectures, tunable circuits and devices.
1.INTRODUCTION
The shortage in the available frequency spectrum for radio communications and the requirement for more functionality in smaller volume increase demand for reconfigurable components. Depending on the device requirements there are different possible solutions like PIN diodes, MEMS or tunable dielectrics to design agile RF components. Regarding high and continuous tunability, low/moderate dielectric losses, high linearity and cost efficiency, liquid crystals (LCs) are promising tunable materials for microwave applications [1]. Tunable microwave devices like patch antennas [2], filters [3] and waveguide structures [4] have been designed and fabricated using LCs. These prototypes also showed that relatively simple and low manifacturing cost make LCs very attractive for commercial applications.
This paper presents the state of the art for LC based reconfigurable antenna arrays: Realized structures and their performances. In the following section, properties of LCs are introduced and dielectric anisotropy of this material is explained for a typical application. In part 3, reconfigurable reflectarrays are discussed for their fabrication, working principle and measurement results. Another type of antennas that is reconfigurable phased array based on
LCs is presented in part 4. For the phased arrays, planar phase shifters are key components. Therefore, different topologies of planar phase shifters are presented and compared, in terms of performances and sizes. Particular attention is given to how LC phase shifters affect critical antenna parameters, like gain, beam steering speed and wide range of scanning. Finally, characteristics of a LC based microstrip patch array are studied.
2.PROPERTIES OF LIQUID CRYSTALS
Depending on the temperature liquid crystal phase exists in a mesophase between a crystalline solid and an isotropic liquid [5] as shown in Fig. 1. In this state, the material can flow like a liquid but at the same time molecules have orientational order. A typical LC molecule has a rod-like shape as shown in Fig. 1. The size of the molecule is typically a few nanometers. This shape anisotropy causes anisotropy in terms of the dielectric constant, as will be described in the following.
Figure 1. Schematic of a typical LC molecule (K15) and its temperature dependency
A cross section of an LC based inverted microstripline (IMSL) is shown in Fig. 2. Similar to LCD technology, there are two substrates for electrodes. The microstripline and ground electrodes are patterned on top and bottom substrates respectively. LC is filled between these two substrates which forms the dielectric of IMSL. For LCs, the average direction of the long axes of the molecules is defined as the director, namely n (Fig. 1). Depending on the RF field distribution and n, LCs feature anisotropic

electrical properties. Thus, LC orientation determines electrical properties of the IMSL. The surfaces of the substrates are coated with a thin polyimide film. This causes to orient LC molecules parallel to the surfaces initially. In this case, the RF field distribution is perpendicular to the director n as shown in Fig. 2b and therefore the relative permittivity and loss tangent along the short axes are effective. These are εr, and tan δ . Molecules can keep their orientation if the applied bias voltage is smaller than the threshold voltage. On the other hand, if the applied voltage exceeds a certain voltage (Vmax) which depends on LC, all molecules are aligned parallel to this bias voltage (Fig. 2c). Then, εr, and tan δ become effective. When the voltage is released again, molecules return to the initial state due to the polyimide film (Fig. 2b). Required time for this process is defined as switching time which depends on LC material, its thickness, temperature and orientation mechanisms. All other states between εr, and εr, , equivalently continuous tunability, can be achieved varying the applied voltage between the threshold voltage and Vmax.
Figure 2. Cross section of an Inverted Microstripline (IMSL)
All LCs studied up to now have positive anisotropy
for |
frequencies |
higher |
than 0.5 GHz, which means |
|
εr |
= εr, − εr, |
> 0, and also for LCs the re- |
||
lationship tan δ |
> |
tan δ holds true. Such a general |
characteristic of LC that is dielectric constant and loss tangent versus bias voltage is plotted in Fig. 3.
3.RECONFIGURABLE REFLECTARRAYS
Liquid Crystal based reconfigurable reflectarrays are studied first by Marin et al. [6] and then in [7, 8, 9] for Kaand lower W-bands. Required technology of a reflecarray fabrication is similar to the IMSL application explained in the previous section. For this manner, instead of fabricating a microstripline on the top substrate,
Figure 3. High frequency εr and tan δ characteristics of LC material versus bias voltage
microstrip patches are realized here as antenna elements. Such a reconfigurable reflectarray is designed based on the principle of variable patch dimensions [10]. Instead of changing the dimensions of the metalized patch, dielectric properties of LC under the patches are tuned with a bias voltage less than 20 V . Hence, although all the patches have identical physical dimensions, they have different electrical properties. This results in different backscattered phases. Beamforming is possible as different path lengths from a feed to the patches are compensated by the preadjusted phases of the patches [8].
One of the realized 1 D-steerable prototypes operating at Ka-band [8] is shown in Fig. 4. It consists of 16 x 16 patches and therefore physical aperture size of the antenna is about 75 mm x 75 mm. The antenna is large enough to obtain relatively narrow beam and a reasonably high directivity. LC cavity is formed by placing spherical spacers with a diameter of 100 μm between two substrates. Although consisting of three dielectric layers which are top substrate, LC layer and bottom substrate (Fig. 2), the prototype has low profile that is less than 10 mm. All elements in a column are connected by a 50 μm thin bias lines, thus all unit cells on one row will provide same phase shift, reducing the number of required bias voltages to 16.
Figure 4. Front view of a realized reflectarray antenna [8]. Antenna size : 75 mm x 75 mm x 10 mm in (x,y,z)
Reflectarrays are first characterized by using two-horn setup [11] in order to extract the bias voltage-phase char-

acteristic of a reflector. The antenna is located in front of two horn antennas which are connected to the ports of a network analyzer. Complex transmission coefficients, namely s21, are measured when all antenna elements are biased with the same control voltage. Reflection coefficients, denoted as s11, can be determined from these measurements. In Fig. 5, continous change of these coefficients are presented for a bias voltage range of 0 V to 20 V . Another possible characterization method is quasioptical setup which is presented in [12].
Figure 5. Reflection coeffcients for different biasing
The power pattern measurements are performed in an anechoic chamber at 36.6 GHz. A feed antenna is located in front of the reflectarray. Illuminated field from the feed antenna is directed to a horn antenna by the reflectarray. In order to illustrate the beam steering ability of the reflectarray, three selected power patterns are presented in Fig. 6 for the E-plane. It should be mentioned that the beam can be scanned continuously form −20◦ to 20◦ (also more). The side lobe level was measured as −5.5 dB at broadside. The reasons for such a low side lobe level are the feed blockage and the multi-reflections between the feed antenna and the reflectarray. Finally, the antenna gain is determined as 15.1 dB.
Figure 6. Measured E-plane power pattern of the antenna
4.RECONFIGURABLE PHASED ARRAYS
Similar to the reflectarrays, reconfigurable phased arrays are of interest due to easy fabrication, low cost, low weight and low profile. Therefore, this part focuses on continous beam steering functionalities of LC based phased arrays.
The main beam of an antenna array is steerable by means of tunable phase shifters. In the framework of Liquida Project which was funded by DLR, LC was combined with Low Temperature Cofired Ceramic (LTCC) technology. With this hybrid technology, an analog LC based inverted microstrip line phase shifter was fabricated [13]. Based on this phase shifter an antenna prototype shown in Fig. 7 was realized.
Figure 7. Realized 4 x 4 reconfigurable patch array (a) Front view (b) Back view
Details of the prototype will be published in another paper. However, measurement results for E-plane are given in order to show the validity of the concept. As shown in Fig. 8, it is possible to steer the main beam from −30◦ to 30◦ in elevation plane with side lobe level lower than −13 dB. Phase shifters and antenna array were fabricated on the same layer in this prototype. Current studies are still going on to design multi-layer reconfigurable phased array architectures. This attempt makes planar phase shifters key components. Hence, different topologies of these phase shifters are presented and compared in the following section.
4.1. Planar Phase Shifters
LC based planar phase shifters have to be customized depending on desired antenna performance. For this manner, different phase shifters have been fabricated for Kaband in order to reduce insertion loss, to increase beam steering speed and to scan wide range.
Receiving antenna figure of merit (G/T) in dB/K can be reduced dramatically when a phase shifter introduces high insertion loss (IL). IMSL type phase shifter as shown in Fig. 9 are preferable since its IL was measured as 0.046 dB/mm at 20 GHz [14]. IL can be reduced
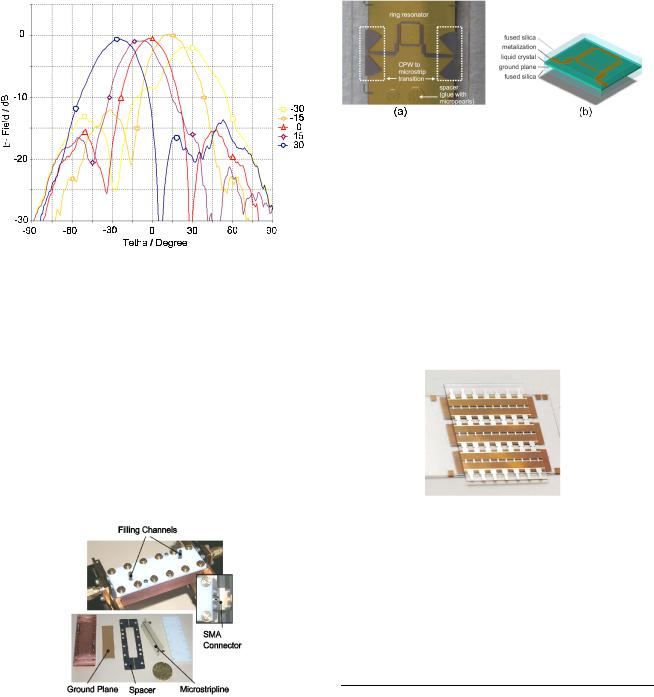
Figure 8. Mesasured E-Plane power pattern of the reconfigurable patch array
even more by increasing LC cavity height whereas this increases switching time of the phase shifter (Table 1). Furthermore, the IMSL topology has less tuning efficiency which is defined as a ratio between achievable differential phase shift ( φ) over phase shifter length with respect to λ0. For instance, for array antennas, one unit cell size is about λ0/2 x λ0/2 . If a meander IMSL type phase shifter is fabricated in such a limited area, the achievable differential phase shift is around 280◦ depending on LC. This limits the scanning range of the antenna. Thus, due to low IL, IMSL phase shifter improves G/T whereas limits beam steering speed and wide range of scanning of an antenna.
Figure 9. Realized IMSL phase shifter
Using a tunable filter [15], i.e. ring resonator as shown in Fig. 10, the tuning efficiency can be increased. The reason for this is the compactness of the ring resonator. Therefore, a phase shifter with diffirential phase shift of 360◦ can be easily fabricated in a limited area by which wide range of scanning becomes possible. On the other hand, being a resonance structure, this topology has high insertion loss. This leads to a degradation in G/T.
A compromise between low insertion loss, fast beam steering speed and wide range of scanning can be
Figure 10. Ring Resonator (a) Photograph from the backside (b) Three dimensional CAD model
achieved using a loaded line phase shifter [16] as shown in Fig. 11. This topology is realized using CPW lines as non-tunable line segments and LC filled parallel plate capacitors as tunable elements. It has two main improvements compared to previous topologies. First, the insertion loss is reduced. This is caused by the fact that the RF field is concentrated on the low loss substrate at the non-tunable segment. Hence, G/T of an antenna can be increased. Second, switching time is reduced due to the thin LC layer of the parallel plate capacitors. Therefore, beam steering speed of an antenna system is increased.
Figure 11. Photograph of Loaded CPW Phase Shifter
Characteristics of these three topologies are given in detail in Table 1. It should be noticed that all three topologies have a similar fabrication process as given in section 2 (Fig. 2). This makes them cost effective when compared to other tunable phase shifter technologies.
Table 1. Comparison of LC Based Planar Phase Shifters
|
IMSL |
Resonator |
Loaded CPW |
Parameters |
[14] |
[15] |
[16] |
|
|
|
|
Frequency / GHz |
20 |
34.3 |
20 |
Lenght / λ0 |
3.3 |
0.23 |
0.87 |
φ / ◦ |
260 |
117 |
120 |
IL / [dB/mm] |
0.046 |
1.91 |
0.12 |
FoM / [◦/dB] |
113 |
30.6 |
60 |
LC layer / μm |
254 |
100 |
4 |
|
|
|
|
LCs have relatively high switching time compared to other tunable device technologies. This switching time
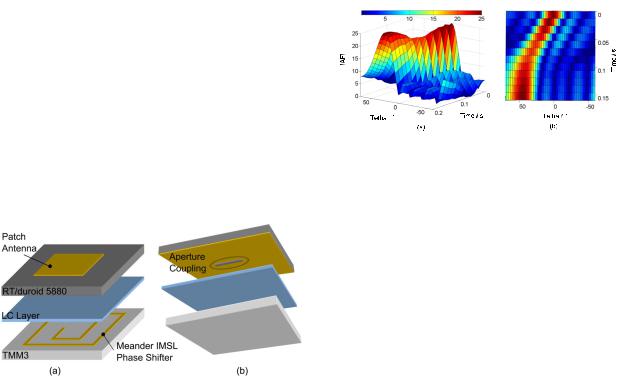
depends on LC material, its thickness, temperature and orientation mechanisms. In general, two main mechanisms are used in order to orient LC molecules. These are mechanically rubbed polyimide film and electrostatic field. The rubbing generates micro-grooves along the rubbing direction [5]. When LC is placed on such a surface, it is aligned parallel to the grooves. This way of alignment increases switching time. For instance, the switching time is measured as 92 ms, for a varactor with a LC layer thickness of 5μm. On the other hand, when the electrostic field is used, the switching time is 22 ms. Beside the orientation mechanism, LC layer thickness has significant impact on the switching time. A varactor with 1μm LC layer thickness requires 4 ms to align LC molecules when the polyimide film is used. This value is reduced to 1 ms if the electrostatic field is used.
4.2. Microstrip Patch Antenna
In this part CST M icrowave Studio simulation results of a reconfigurable microstrip patch antenna are presented for Ka-band. An antenna consists of three dielectric layers is designed. These layers are RT/duroid 5880, LC and TMM3 as shown in Fig. 12. There are 25 x 25 patch elements and therefore the overall size of the antenna is 20 cm x 20 cm x 1 cm. Meander IMSL phase shifters with 360◦ differential phase shift are used and they are coupled to the radiating elements via aperture coupling. Bandwidth and radiation efficiency of one unit cell are determined as 6 % and −0.62 dB, respectively. The antenna gain is about 25 dB and the main beam direction can be scanned ± 60◦ in elevation plane in the absence of mutual coupling.
Figure 12. Expanded views of one unit cell (a) Topperspective view (b) Bottom-perspective view
It is a challenge to design an antenna architecture where LNAs are placed between antenna elements and phase shifters using LC technology. Therefore, by assuming that the LNAs are located behind the phase shifters, G/T can be calculated to 1.6 dB/K for a system with the following values: array gain = 30 dB, antenna noise temperature = 50 K, phase shifter insertion losses = 5 dB, ambient temperature = 290 K, LNA gain = 20 dB and LNA noise figure = 4 dB. All expenses of such a system is mainly determined by the cost of LNAs. The cost of the antenna and phase shifters can be compared with that of a LCD which is 20 cm x 20 cm in size.
Effects of the high LC switching time on the antenna performance have to be studied. Optimizations on beam forming algorithms and phase distribution over the antenna can be performed. However, significant improvement has been achieved by customizing phase shifter topologies. For instance, the beam steering speed is around 1 ◦/s empirically if IMSL type phase shifters are used. This value can be increased up to 200 ◦/s and higher using a CPW loaded line phase shifter because of the thin LC layer (Table 1).
Array factor (AF) over time is given in Fig. 13 for a scenario of steering the main beam from 0◦ to 45◦ in elevation plane. Although an optimum AF with high directivity and high side lobe level can be achieved after 0.16 s, the antenna can still get signal from 45◦ even after 0.1 s. In this case, the side lobe level is lower than the optimum value because the phase shifters have not reached their desired differential phase values.
Figure 13. Array factor (AF) of a 5 x 5 antenna array over the time (a) Perspective view (b) Top view
5.CONCLUSION AND DISCUSSION
This paper presents the fundamentals of LC material and its applications for reconfigurable antenna arrays like reflectarrays and phased arrays. The most critical issue was the dielectric loss tangent of this material which was reduced with interdisciplinary cooperations with materials scientists and chemists. Achieved results up to now show that LC is a promising material for microwave applications in terms of device performance and cost. Reconfigurable reflectarray was presented for Ka-band (and also fabricated for W-band). These antennas are suitable and compact solutions for satellite antennas due to their planarity, low mass and low manufacturing cost with respect to parabolic reflector. For end user antennas, i.e. automotive electronically steerable antennas being integrated onto a car body, it is preferable to use phased arrays due to low profile. Tunable planar phase shifters are significant for multi-layer phased arrays. Thus, these phase shifters are discussed in details in order to figure out how they affect antenna performances like G/T, beam steering speed and wide range of scanning. LC technology is a cost effective solution for commercial microand mmwave tunable devices because the required technology for fabrication of these devices is relatively simple as other technologies.
ACKNOWLEDGMENTS
The authors wish to acknowledge to CST Company for providing the software. Presented works were supported by German Research Foundation DFG within the Research Training Group 1037 ”Tunable integrated components in microwave technology and optics” (TICMO). We would also like to thank DLR for funding Liquida Project and all other partners for useful discussions.
REFERENCES
[1]Karabey, O.H., Goelden, F., Gaebler, A. and Jakoby, R., ”Precise Broadband Microwave Material Characterization of Liquids,” Proc. of 40th EuMC 2010, Paris
[2]Luyi Lui, Richard Langley ”Electrically Small Antenna Tuning Techniques,” Lounghborough Antenna and Propagation Conference, 2009
[3]Goelden, F., Gaebler, A., Karabey, O., Goebel, M., Manabe, A. and Jakoby, R., ”Tunable Band-pass Filter Based on Liquid Crystal,” Proc. of German Microwave Conference, Berlin, 2010
[4]Gaebler, A., Goelden, F., Manabe, A., Goebel, M., Mueller, S. and Jakoby, R., ”Investigations on High Performance Transmission Line Phase Shifters Based on Liquid Crystal,” Proc. of 39th EuMC 2009, Rome
[5]Yang, D.-K., Wu, S.-T., Fundamentals of Liquid Crystal Devices, (2006) Wiley & Sons
[6]Marin R., Moessinger A., Freese, J., Mueller S., Jakoby R., ”Basic Investigations of 35 GHz Reflectarrays and Tunable Unit-Cells for Beamsteering Applications,” Proc. of 35th EuMC 2005, Paris
[7]Moessinger A., Marin R., Freese J.,Mueller S.,Manabe A., and Jakoby R., ”Investigations on 77 GHz Tunable Reflectarray Unit Cells with Liquid Crystal,” Proc. of EuCAP 2006, (ESA SP-626, October 2006), France
[8]Bildik S., Fritzsch C., Moessinger A., Jakoby R., ”Tunable Liquid Crystal Reflectarray with Rectangular Elements,” Proc. of German Microwave Conference, Berlin, 2010
[9]Ismail M.Y., and Zain A.F.M., ”Phase Tunability of Reflectarray Patch Elements Using Tunable Dielectric Substrate of Nematic Liquid Crystal,” IEEE International Workshop on Antenna Tech., Santa Monica, 2009
[10]Pozar, D.M., Targonski, D., Syrigos, H.D., ”Design of Millimeter Wave Microstrip Reflectarrays,” IEEE Trans. on Antenna and Prop., vol. 45, no. 2, 1997
[11]Marin, R., Moessinger, A., Goelden, F., Mueller, S., Jakoby, R., ”77 GHz Reconfigurable Reflectarray with Nematic Liquid Crystal,” Proc. of EuCAP 2007, Edinburg
[12]Moessinger A. , Dieter S., Menzel W., Mueller S., and Jakoby, R., ”Realization and Characterization of a 77 GHz Reconfigurable Liquid Crystal Reflectarray,”
13th International Symposium on Antenna Technology, Canada, 2009
[13]Rittweger, M., Kulke, R., Follmann, R., Wolff, I, ”Innovative Technologies for RF Circuitry in Satellite Payload,” 3rd European Conference on Antennas and Propagation, Berlin, 2009
[14]Mueller, S., ”Grundlegende Untersuchengen steuerbarer passiver Fluessigkristall-Komponenten fuer die Mikrowellentechnik,” D17, pp.104-115, Shaker Verlag 2007, Aachen
[15]Moessinger, A., Fritzsch, C., Bildik, S. and Jakoby, R., ”Compact Tunable Ka-Band Phase Shifter based on Liquid Crystals,” Proc. of IMS, Anaheim, 2010
[16]Goelden, F., Gaebler, A., Goebel, M., Manabe, A, Mueller, S. and Jakoby, R., ”Tunable Liquid Crystal Phase Shifter for Microwave Frequencies,” Electronic Letters, vol. 45, no. 13, 2009
View publication stats