
диафрагмированные волноводные фильтры / 362aab6a-244b-49df-bac0-ae2c085694d9
.pdf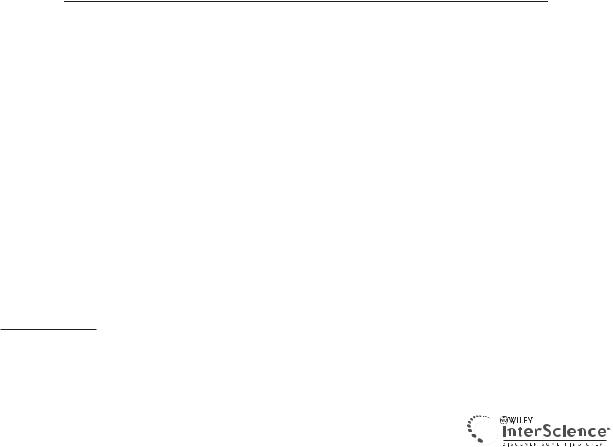
Miniaturized Rectangular Waveguide Filters
Alexander Shelkovnikov, Djuradj Budimir
Wireless Communications Research Group, Department of Electronic, Communication and Software Engineering, University of Westminster, London W1W 6UW, United Kingdom
Received 11 May 2006; accepted 20 September 2006
ABSTRACT: Two types of miniaturized rectangular waveguide filters are presented. Miniaturization is achieved using the slow-wave effect of electromagnetic bandgap (EBG) surfaces and the left-handed properties of split ring resonators (SRRs). The proposed EBG waveguide bandpass filter performs passband in the frequency range, which corresponds to the waveguide with the lower recommended operating band consequently enabling significant miniaturization of the structure. The SRR-loaded bandstop filter makes use of the effect imposed by left-handed medium (LHM), which is created by a combination of SRRs and wireline on the dielectric slab. Both filters are designed, simulated, and tested. Experimental results of the SRR-loaded bandstop filter are presented to demonstrate feasibility of the proposed structures.
Keywords: electromagnetic bandgap (EBG) structures; left-handed medium (LHM); rectangular waveguides; split ring resonators (SRRs); waveguide filters
I. INTRODUCTION
The explosive growth in commercial interest in RF, microwave, and millimeter-wave systems, especially in wireless communications and mobile and satellite communication systems has provided a significant challenge to conventional microwave circuits and their design methodologies. High performance nar- row-band bandpass filters having a low insertion loss, compact size, wide stopband, and a high selectivity are important for next-generation wireless systems. At present most filters at RF, microwave, and milli- meter-wave frequencies are produced either in waveguide (air-filled metal pipe, dielectric-filled or micromachined air-filled) with high associated machining costs, image guide, and nonradiative dielectric guide
Correspondence to: D. Budimir; e-mail: d.budimir@ westminster.ac.uk
DOI 10.1002/mmce.20238
Published online 25 June 2007 in Wiley InterScience (www. interscience.wiley.com).
with high associated loss or using dielectric resonators or using printed circuit board (PCB) and multilayered low-temperature-cofired-ceramics (LTCC) technologies. Planar and quasi-planar guiding structures (microstrip, suspended substrate stripline, and coplanar waveguide) are the common choices for MIC circuit designers in the multi-layered LTCC and PCB. Generally these filter structures are not able to achieve high selectivity characteristics because the Q values are limited by loss and radiation. Although in principle highly selective characteristics can be achieved by using elliptic function filters, this technique becomes less viable at mm-wave frequencies.
Rectangular waveguides have been a sustainable solution over the past few decades, used to design robust, low loss and high power circuits at microwave and mm-wave frequencies. The classical rectangular waveguide theory is still very much usable in order to build various filter structures, which are viable to meet requirements of the modern technology [1–3]. However, reduction of the physical size of such structures has become one of the primary goals.
VC 2007 Wiley Periodicals, Inc.
398

Figure 1. Configuration of a conventional rectangular waveguide bandpass filter.
Recently proposed concepts of electromagnetic bandgap (EBG) and left-handed medium (LHM) have become the subject of extensive investigations due their capability to provide novel unconventional properties to different propagation media [4, 5]. The first approach relies on the theory of high-permittivity surfaces, also known as high-impedance electromagnetic surfaces [4]. They are typically constructed as a piece of dielectric substrate with an array of small metal patches printed on top connected the bottom by metal plated via-posts, and where the bottom plane serves as a metal ground. The second approach makes use of the LHM created by a novel type of resonance elements, split ring resonators (SRRs), in combination with the thin metal wireline [5]. These are printed on the dielectric slab, which is then inserted into the plane of symmetry of the rectangular waveguide.
Both such structures are able to alter the electromagnetic boundary conditions of the surface and prohibit propagation of signal in a certain frequency band. Thus, the traditional miniaturization techniques, which commonly employ dielectric-filled waveguides with standard dimensions bound to the wave-
Figure 2. Configuration of EBG waveguide bandpass filter.
Miniaturized Rectangular Waveguide Filters |
399 |
TABLE I. Dimensions of the EBG Waveguide |
|
Bandpass Filter |
|
|
|
Parameter |
(in mm) |
|
|
Length of square metal patches, lp |
2.0 |
Diameter of copper via-posts |
0.25 |
Length of metal septa, ls1 ¼ ls3 |
1.4 |
Length of metal septum, ls2 |
4.0 |
Length of resonator sections, lr1 ¼ lr2 |
7.0 |
Total length of the waveguide filter, Lwg |
25.8 |
length (k), may be enhanced to achieve more compact high-performance waveguide components.
In this article, we make use of the slow-wave effect provided by the high-impedance surfaces and the left-handed properties imposed by SRRs in order to achieve miniaturization of rectangular waveguide filters.
II. EBG WAVEGUIDE BANDPASS FILTERS
A. Design of the Proposed
Configurations
The standard E-plane filter is realized by coupling the half wavelength resonators through K-inverter prototype. Values for the K-inverter are obtained through the performance of metal inserts as proposed in [6], allowing for negative length of the transmission line. The distance between successive metal-inserts for the filter is then kg/2, reduced by the negative lengths of the K-inverter. Following the relation of the resonator length to the guided wavelength, the slow-wave effect of the EBG surfaces incorporated in the waveguide can be used in order to reduce the size of an E-plane filter. Figure 1 shows configuration of the conventional rectangular waveguide filter.
The EBG waveguide bandpass filter design employs the standard metallic waveguide (WG-19: a ¼ 12.954 mm, b ¼ 6.477 mm) in order to incorporate four high-impedance surfaces on the top and bottom planes of both resonator sections of the filter. The high-impedance surface is constructed from a 0.508- mm-thick (h) RT/Duroid dielectric substrate (er ¼ 2.2) with an array of small, much smaller than the wavelength, square-shaped metal patches printed on the top plane [7]. Each of the metal patches is connected to the bottom ground surface of the dielectric through a transversally drilled copper via-post. Configuration of the proposed EBG waveguide bandpass filter is illustrated in Figure 2. Dimensions of the waveguide filter are presented in Table I.
International Journal of RF and Microwave Computer-Aided Engineering DOI 10.1002/mmce

400 Shelkovnikov and Budimir
Figure 3. Insertion loss of the EBG waveguide bandpass filter comparing to the conventional rectangular waveguide prototype.
Figure 5. Variation of resonance behavior of SRRloaded waveguide unit with lengths of metal septa (ls ¼
ls1 ¼ ls2).
B. Subwavelength Performance
To validate the argument the EBG waveguide bandpass filter is simulated and compared with its conventional rectangular waveguide prototype. The threedimensional finite-element-based commercial electromagnetic simulator was used for simulation [8].
Results of simulation are presented in Figure 3. It is evident that the passband of the filter, which employs the slow-wave effect imposed by the EBG waveguide, is shifted towards the lower operating frequency band. This breaks the k/2 limitation and enables significant reduction of physical dimensions of the waveguide comparing to standard rectangular waveguide filters. Moreover, introduction of EBG enables improvement of the Q factor. For the designed EBG waveguide resonator QL ¼ 172.
III. SRR-LOADED WAVEGUIDE
BANDSTOP FILTERS
A. SRR-Loaded Waveguide Resonator
Units
Design of the SRR-loaded rectangular waveguide is initially based on the concepts of a three-dimensional (3D) composite medium unit cell, which can be created by the interaction of electrically small resonant particles with the thin conducting wires [5]. Arranging both the SRR and the metal wireline together on the same dielectric substrate and placing centrally into a hollow metallic waveguide, we can realize an SRR-loaded waveguide unit that supports similar properties of the 3D composite medium. Furthermore, in order to control the effect we propose to embed a set of additional transversely-placed metal septa within each of the unit cells. Such configuration
Figure 4. Configuration of SRR-loaded waveguide reso- |
Figure 6. Configuration of SRR-loaded waveguide band- |
nator unit. |
stop filter. |
International Journal of RF and Microwave Computer-Aided Engineering DOI 10.1002/mmce

TABLE II. Dimensions of the SRR-Loaded
Bandstop Filter
Parameter |
(in mm) |
|
|
Thickness of the wireline, twl |
0.2 |
Length of metal septa, ls1 ¼ ls3 |
0.2 |
Length of metal septum, ls2 |
1.0 |
Length of resonator sections, lr1 ¼ lr2 |
8.0 |
Total length of the waveguide filter, Lwg |
20.4 |
of the SRR-loaded waveguide unit is able to perform controlled resonance at the desired frequency. Illustration of the SRR-loaded waveguide resonator unit is shown in Figure 4.
The SRR-loaded waveguide resonator unit is constructed by fitting the discontinuities in form of metallic septa on the dielectric slab. To illustrate the effect of lengths of metallic septa on the resonant behavior of the waveguide unit, the substrate is fitted with septa ls1 ¼ ls2 ¼ 2.2-mm-long. Reducing the length of metallic septa (ls1,2), we control the shift of the resonant frequency and achieve improvement of the Q factor of the resonator unit (QL ¼ 125). Variation of the resonance behavior with the lengths of metal septa is illustrated by the simulated transmission coefficients in Figure 5.
B. SRR-Loaded Waveguide
Bandstop Filter
The SRR-loaded waveguide bandstop filter is realized as a cascade of the resonator unit cells. The transmission line is loaded with the slab of a composite mate-
Miniaturized Rectangular Waveguide Filters |
401 |
Figure 8. Simulated and measured insertion loss of the SRR-loaded waveguide bandstop filter.
rial, which conveniently facilitates both SRRs with the metal septa on the top plane, and a thin wireline stretched throughout the full length of the dielectric on its bottom plane. Configuration of the SRR-loaded waveguide bandstop filter is shown in Figure 6.
SRRs are characterized by the following parameters: ring thickness (s ¼ 0.25 mm), inner diameter (w0 ¼ 2.62 mm), and ring gap (g ¼ 0.48 mm). The filter was designed and simulated with its dimensions presented in Table II.
To illustrate the feasibility of the proposed structure the SRR-loaded waveguide bandstop filter has been fabricated and tested at X-band. Standard rectangular waveguide (WG-16: a ¼ 22.86 mm, b ¼ 10.16 mm) has been used as a housing to fit a 0.508-mm- thick dielectric slab. As illustrated in Figure 7, the
Figure 7. Measured transmission and reflection coefficients of the SRR-loaded waveguide bandstop filter.
Figure 9. Photograph of the fabricated bandstop filter together with the waveguide housing.
International Journal of RF and Microwave Computer-Aided Engineering DOI 10.1002/mmce

402 Shelkovnikov and Budimir
filter performs a wide stopband of 1 GHz, which can be controlled by tuning the resonance of SRRs and lengths of metal discontinuities. The measured response demonstrates agreement with the simulation (see Fig. 8). Total length of the filter including waveguide feeding is 20.4 mm (see Fig. 9), which represents a 108% reduction of the filter size comparing to the conventional E-plane waveguide filter. The filter can be modified further to provide passband bandpass within the required frequency range by changing configuration of the rings.
IV. CONCLUSIONS
Two types of miniaturized rectangular waveguide filters have been presented. The EBG waveguide bandpass filter has been designed, simulated and compared with the standard E-plane bandpass filter. Because of the slow-wave effect of high-impedance surfaces, the proposed filter is able to break the k/2 limitation and perform at subwavelengths. This enables the possibility of a significant size reduction. The SRR-loaded waveguide bandstop filter has been designed, simulated, and measured. Using the combination of the SRRs, conductive wireline, and transversely placed metal septa, the composite material has been built and inserted into the hollow rectangular waveguide to form an SRR-loaded waveguide unit. The stopband filter has been constructed by cascade of the two resonator unit cells. The filter has
been fabricated and tested, showing the feasibility for application in microwave communication systems.
REFERENCES
1.V. Postoyalko and D. Budimir, Design of waveguide E-plane filters with all-metal inserts by equal-ripple optimization, IEEE Trans Microwave Theory Tech MTT-42 (1994), 217–222.
2.F. Arndt, The status of rigorous design of millimeter wave low insertion loss fin-line and metallic E-plane filters, J Instn Electron Telecom Eng 34 (1988), 107– 119.
3.J. Uher, J. Bornemann, and U. Rosenberg, Waveguide components for antenna feed systems: Theory and CAD, Artech House, Norwood, MA, 1993.
4.D. Sievenpiper, L. Zhang, R. Broas, N. Alexopolous, and E. Yablonovitch, High-impedance electromagnetic surfaces with a forbidden frequency band, IEEE Trans Microwave Theory Tech 47 (1999), 2059–2074.
5.D.R. Smith, W.J. Padilla, D.C. Vier, S.C. NematNasser, and S. Schultz, Composite medium with simultaneously negative permeability and permittivity, Phys Rev Lett 84 (2000), 4184–4187.
6.D. Budimir, Optimized E-plane bandpass filters with improved stopband performance, IEEE Trans Microwave Theory Tech 45 (1997), 212–220.
7.A. Shelkovnikov and D. Budimir, Novel compact EBG waveguide resonators in planar form, Proc. 35th European Microwave Conference (EuMC’2005), Paris, France, October, 2005.
8.High-Frequency Structure Simulator, v.9.0, Ansoft Corporation, 2003.
BIOGRAPHIES
Alexander Shelkovnikov received BEng. degree in electronic engineering and MSc. degree in electronic and telecommunication systems, both from the National Technical University of Ukraine, Kiev, Ukraine, and Ph.D. degree in electronic engineering from the University of Westminster, London, UK. He was with the Institute of Telecommunication Systems
at the National Technical University of Ukraine from 1999 to 2002. In October 2002 he joined the Wireless Communications Research Group, University of Westminster, where he is currently involved with the design of new microwave integrated circuits and waveguide components such as filters, diplexers and multiplexers for wireless communication systems, and electromagnetic analysis and design of EBG and SRR loaded waveguide filters for microwave and millimeter-wave applications.
Djuradj Budimir was born in Serbian Krajina formerly Yugoslavia. He received the Dipl. Ing. and MSc. degrees, both in Electronic Engineering from the University of Belgrade, Belgrade, Serbia, and Ph.D. degree in Electronic and Electrical Engineering, University of Leeds, Leeds, UK. In March 1994, he joined the Department of Electronic and Electrical Engineering at
Kings College London, University of London. Since January 1997, he has been with the Department of Electronic, Communication and Software Engineering, University of Westminster, London, UK. He is now an Associate Professor with the Department of Electronic, Communication, and Software Engineering, University of Westminster, London, UK. His research interests include analysis and design of hybrid and monolithic microwave integrated circuits, the design of amplifiers, filters and multiplex-
International Journal of RF and Microwave Computer-Aided Engineering DOI 10.1002/mmce
ing networks for RF, microwave and millimetre-wave applications and RF and microwave design of wireless systems. Dr Budimir has written over 130 refereed journals including IEEE Trans. on Microwave Theory Techniques, IET Proc. Microwaves, Antennas and Propagation, IEEE Microwave and Wireless Components Letters, IET Electronics Letters and Microwave Technology Optical Letters, and conference proceeding papers in the field of RF, microwave and millimetre-wave circuits. He is author of the book Generalized Filter Design by Computer Optimization (Artech House, 1998) and Software and Users
Miniaturized Rectangular Waveguide Filters |
403 |
Manual EPFIL-Waveguide E-plane Filter Design (Artech House, 2000), and a book chapter in the book ‘‘Encyclopaedia of RF and Microwave Engineering,’’ Wiley, February 2005. He is the owner of software (DBFILTER). He is a senior member of IEEE, member IEE and a Chartered Engineer. He is also a regular referee for IEE Electronic Letters, IET Proceedings Microwaves, Antennas, and Propagation, IEEE Microwave and Wireless Components Letters, IEEE Transactions on Microwave Theory and Techniques, IEEE Transactions on Circuits and Systems.
International Journal of RF and Microwave Computer-Aided Engineering DOI 10.1002/mmce